[Image above] Illustration showing the heat flow at a sharp leading edge on a hypersonic or space vehicle protected by a passive thermal protection system. Energy is transferred into the body via conduction and/or transferred back to the environment by radiation. Credit: Saad et al., International Journal of Ceramic Engineering & Science (CC BY 4.0)
The pursuit of space travel has experienced a resurgence in recent years, and with it the launch of programs and funding initiatives to develop the materials and technologies necessary to achieve this dream. But it is not only new paradigms that are being explored—in some cases, such as ultrahigh-temperature ceramics (UHTCs), it is realizing the potential of an existing material class.
UHTCs are refractory ceramics with the formulation M–X, where M is an early transition metal (groups 4–5 of the periodic table) and X is either a boron, carbon, or nitrogen. They have very high melting temperatures (>3,000°C) as well as other useful thermomechanical properties.
The U.S.–Soviet Union space race in the mid-20th century kicked off systematic study of UHTCs, but today’s commercial applications of these materials are in other sectors, such as aluminum production and boiling water reactors. Researchers are hopeful, though, that this recent focus on space research will allow UHTCs to finally fulfill their potential and help us travel to the stars.
The American Ceramic Society is doing its part to educate the public about UHTCs. Last week, the Society announced that it received a contract from the Department of Defense Cornerstone Consortium to develop a workforce training program on the science and engineering of materials for hypersonic applications, including UHTCs. ACerS is partnering with the United States Advanced Ceramics Association on the effort.
The first course under this program took place at the Composites, Materials & Systems Conference in St. Augustine, Fla., on Jan. 21, 2024. ACerS Fellow and Purdue University professor Rodney Trice taught the one-day short course.
Trice has focused on many fundamental and applied research topics over the years, but the high-temperature properties and processing of ceramics is one area in which he specializes. For example, he and his students have published numerous papers on high-emissivity materials to protect the leading edges of hypersonic and space vehicles during atmospheric re-entry.
Emissivity describes how efficiently a material can radiate thermal energy. A high emissivity (with a value close to 1) maximizes heat radiation from a hot surface, such as an aircraft’s leading edges.
Researchers have studied the radiative behavior of various UHTC systems to identify ones that could serve as high-emissivity coatings for hypersonic vehicles and spacecraft. Intrinsic and extrinsic methods to improve the emissivity of UHTCs have also been investigated.
Last year, Trice published an open-access review paper with Purdue associate professor Carlos Martinez and graduate student Abdullah A. Saad summarizing these UHTC emissivity studies. Highlights from the 23-page paper are below.
Radiation distribution and the four types of emittances
The first section of the paper familiarizes readers with the concepts of thermal radiation and emissivity. One key point is understanding how the wavelength and direction of radiation affect its distribution from a surface.
“Radiation emitted from an opaque surface at a specific orientation typically spans a range of wavelengths … The wavelength-dependent distribution of radiation is referred to as a spectral distribution. In addition, an opaque surface may radiate preferentially in a particular direction, creating a directional distribution of emitted radiation,” the authors write.
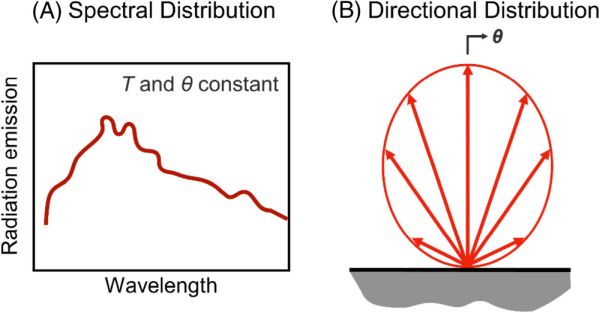
Figure 4 from the paper shows how radiation can be emitted from a surface as (A) spectral distribution and (B) directional distribution. Credit: Saad et al., International Journal of Ceramic Engineering & Science (CC BY 4.0)
Based on the category of radiation distribution, there can be four types of emittances: spectral emittance, total emittance, directional emittance, and hemispherical emittance. In practice, emittances defined by the spectral distribution (first two types) are coupled to those defined by the directional distribution (last two types). Measuring all four types of emittances at high temperatures is essential to determine the radiative cooling potential of various UHTCs.
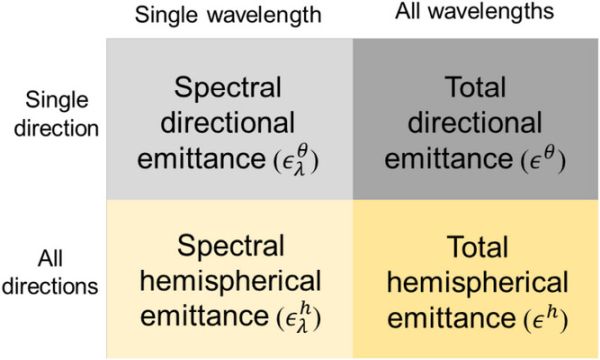
Figure 5 from the paper shows the relationship between wavelength-dependent emittances and direction-dependent emittances. Credit: Saad et al., International Journal of Ceramic Engineering & Science (CC BY 4.0)
Measuring emittances
The emittance of a surface can be measured directly or indirectly through radiometric or calorimetric methods, respectively.
In the direct (radiometric) method, the emitted energy from a sample is measured directly using sophisticated optical instruments, such as Fourier transform infrared spectroscopy. The results are subject to systematic error due to the complexity and uncertainty augmented by multiple instruments involved in this technique.
In the indirect (calorimetric) method, the emitted energy from a sample is measured indirectly using a calorimeter. Unlike the radiometric method, the calorimetric method does not yield wavelength-specific or direction-specific radiative thermo-optical properties. On the other hand, it produces fewer erroneous results when testing at high temperatures.
Because both radiometric and calorimetric methods can be expensive to set up and operate at high temperature, some researchers have adopted an inexpensive emission measurement technique based on the principle of two-color pyrometry to estimate the emittances at high temperatures.
However, this method depends on correctly estimating various ratios of multiple variables as well as correctly maintaining a consistent direction of the pyrometers during testing. As such, it does not always provide accurate results, so emittance values measured this way cannot be used as a standard reference.
Enhancing UHTC radiative properties
In the paper’s fourth section, the authors provide a table that lists the emittance values for various UHTCs determined through past radiometric and calorimetric studies. Then, they discuss several emittance enhancing techniques reported in the literature to improve the radiative properties.
For example, borosilicate layer-induced enhancement is commonly found in emittance studies of ZrB2-SiC-based composites. The SiO2-rich borosilicate layer formed through oxidation of ZrB2-SiC can effectively improve emittance of the surface in the temperature range 1,100−1,200°C.
Additionally, rare earth oxides and rare earth ions are known for their high intrinsic emissivity in the infrared spectral region at high temperatures. Incorporating these materials into UHTCs can enhance emittance in the temperature range 1,200–1,600°C.
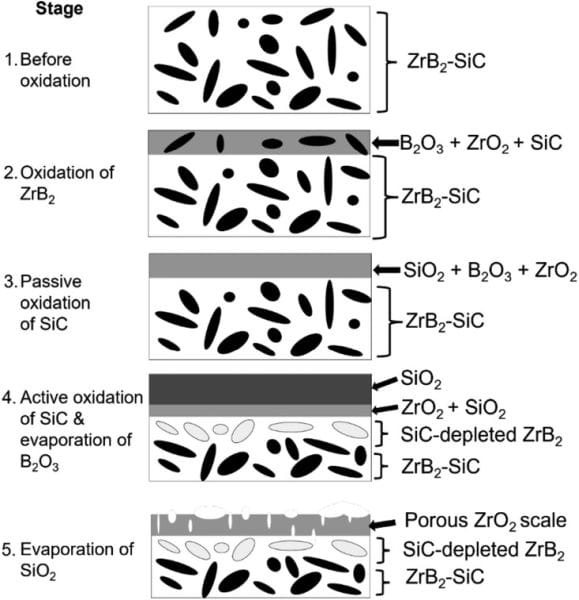
ZrB2-SiC oxidation reaction sequence based on the model proposed by Fahrenholtz (2006) and other experimental observations. Emittance starts to rise during borosilicate formation (stage #3) and drops after complete evaporation of SiO2 (stage #5). Credit: Saad et al., International Journal of Ceramic Engineering & Science (CC BY 4.0)
Finally, microstructure-induced emittance modification is also possible, as Wang et al. demonstrated in a 2015 paper. They compared the radiative performance of spherical SiC particles and longitudinal SiC whiskers used as a secondary phase in a ZrB2–20 vol.% SiC composite microstructure up to 1,600°C. The low surface-area-to-volume ratio of whisker geometry resulted in enhanced thermal radiation.
Several other methods were also discussed, including the use of sintering additives, incorporating SiC fibers as a secondary phase, and forming UHTC matrix composites.
Future directions
The authors conclude the paper by suggesting several future directions for research. For example, they note that there is no universally accepted standard method for conducting high-temperature radiometric measurements.
“Further research is needed to design an in-situ standardized, precision emissometer so that the emittance can be measured in real-time, along with oxidation testing at temperatures over 1,800°C,” they write.
Additionally, knowledge of emittance values at temperatures of more than 1,800°C is currently lacking, so methods for conducting experiments at those temperatures are needed.
Ultimately, the lessons drawn from this study should allow scientists “to measure and enhance the emissivity of UHTC materials for hypersonic applications,” the authors write.
The open-access paper, published in International Journal of Ceramic Engineering & Science, is “Radiation heat transfer during hypersonic flight: A review of emissivity measurement and enhancement approaches of ultra-high temperature ceramics” (DOI: 10.1002/ces2.10171).
Author
Lisa McDonald
CTT Categories
- Aeronautics & Space
- Education
- Thermal management