[Image above] Charles T. McLaren (left) and Himanshu Jain create alkali silicate glass for their research. Alkali silicate glass transforms from homogeneous to inhomogeneous upon exposure to an electric field, and thus its heating cannot be predicted by traditional Joule’s first law. Credit: Lehigh University
We usually do not notice how much we rely on consistency in our lives—until a pesky stair a little taller than its companions causes us and countless others to trip. While viral stair-tripping videos provide one example of the consequences of failed consistency, stairs are far from the only product that we expect consistency from to ensure everything runs smoothly.
For example, scientists rely on consistency of equations to perform successful experiments. When plugging numbers into a mathematical formula, we generally expect experimental results to (closely) match calculated values. When theoretical and experimental values do not match, it can be cause for confusion, especially when the equation used to calculate theoretical values has been trusted for decades or centuries.
One well-known equation is Joule’s first law of heating, which has been around since the 1840s. According to this law, heat is produced in proportion to the square of electrical current passing through a material. The reliability and validity of this law is readily confirmed when calculating values for homogeneous conductors or semiconductors. For inhomogeneous materials like composites, scientists have extended the law by restating that heat is produced in proportion to the square of the local electrical field.
And then there are alkali silicate glasses.
Alkali silicate glasses are homogeneous materials, so one would expect them to heat uniformly according to Joule’s law. However, upon exposure to an electric field, the local temperature near the anode reaches temperatures more than 1,000ºC hotter than the rest of the glass—well above the predicted Joule temperature for homogeneous materials! We know Joule’s first law works, so what is causing alkali silicate glasses to supposedly defy this respected law?
In a new open-access paper by Lehigh University and Corning Inc. researchers, the answer is surprisingly simple—as alkali silicate glasses heat, they become inhomogeneous on the nanoscale and thus do not fit the requirements necessary to be predicted by traditional Joule’s law.
“Trivial expressions of Joule heating are well-established,” the researchers say in the paper. “However, much less is known about the manifestation of Joule’s law for the case of a material that is homogeneous to begin with, but wherein the effect itself (by migrating charge-carriers) may modify the material over time.”
The researchers decided to study homogeneous-to-inhomogeneous material transformation in alkali silicate glass because of a recently discovered phenomenon in model alkali silicate glasses: electric field induced softening (EFIS).
EFIS was first reported by Lehigh University and University of Colorado at Boulder researchers in 2015. This mechanism softens glass at much lower furnace temperatures than conventional heating methods, making it easier to fabricate different structures in glass, such as microlenses.
By studying EFIS in alkali silicate glass, the researchers hoped to both better understand EFIS and how Joule’s first law may need to be modified to accurately depict other cases where materials change structure under an applied electric field, such as thin film and nanoscale devices (devices in which inhomogeneity becomes destructive more readily).
For their study, the Lehigh and Corning researchers created two lithium sodium silicate glasses and a sodium silicate glass to serve as models of common glasses. Using representative infrared images, the researchers identified four stages of glass heating that occur when an electric field is applied (building on research from a previous study).
Steps to heating
(a) The glass begins to resistively heat locally near the anode much more than anywhere else.
(b) Positive feedback of thermal runaway builds up, quickly causing intense heating of the glass near the anode.
(c) Sparks occur near the anode and sometimes within the graphite anode.
(d) The glass begins to soften locally and the generated heat diffuses into the bulk sample by thermal conduction, thereby extending softening/deformation to the rest of the sample.
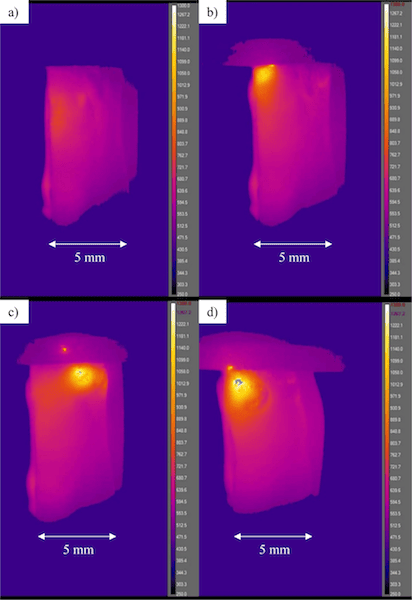
Credit: McLaren, Kopatz, Smith, and Jain; Scientific Reports (CC BY 4.0)
Scientists have largely attributed the EFIS process to formation of a highly resistive alkali ion depletion layer near the anode, which leads to dielectric breakdown (the glass goes from being insulating to electrically conductive) and thermal runaway (an increase in temperature causes a further increase in current and thus temperature).
However, whether dielectric breakdown causes thermal runaway or thermal runaway causes dielectric breakdown is still an open question. As the researchers say, “The present results may not fully resolve this dilemma of cause and effect between dielectric breakdown and thermal runaway.”
Though this study could not conclusively answer this specific technicality, the researchers did shine light on part of the EFIS process: thickness of the alkali ion depletion layer influences extent of thermal runaway.
“We had no purpose of controlling [the depletion layer’s] thickness,” says Himanshu Jain, ACerS fellow and professor of materials science and engineering at Lehigh University, in an email. “Formation of depletion layer … is induced by the application of electric field. The depletion layer develops and grows with time until reaching its stable value.” However, by observing what happened at each thickness as the depletion layer grew, the researchers were able to gather some insights.
“The maximum temperature experienced by the depletion layer was when its thickness was 100 nm,” the researchers explain in the paper. At 100 nm thickness, they found local temperature increased by about 1,400°C in less than 30 seconds, while the furnace temperature increased by only 10.1°C!
In contrast, when depletion thickness was very small (like 5 nm), the generated heat “could dissipate quickly out into the electrode by conduction” and thus avoid thermal runaway, while thickness beyond 100 nm began to limit the amount of current that could pass through, and thus “the reduced current decreased the attendant Joule heating effects.” Once depletion layer thickness reached 50 μm, there was no indication of thermal runaway and the sample “represents the condition of classic Joule’s law.”
Importantly, these results occurred when using DC applied voltage. The researchers found “resistive heating in AC-EFIS could be more controllable compared to the dramatic thermal runaway of DC-EFIS, likely due to a more uniform internal field and corresponding heat distribution under AC than in the analogous DC case.”
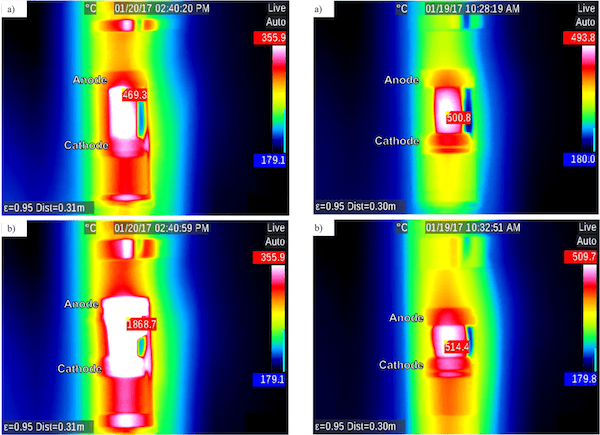
Infrared images showing heating under DC (left) and AC (right) current. Hottest pixel is identified with surface temperature reading. In the DC condition, furnace temperature rose from 353.5°C to 363.6°C and sample temperature increased over 1,000°C. In the AC condition, furnace temperature rose from 385.3°C to 428.6°C and sample temperature increased about 14°C. Credit: McLaren, Kopatz, Smith, and Jain; Scientific Reports (CC BY 4.0)
While this study showed macroscale Joule’s law for homogeneous samples does not apply to ionically conducting solids when metal or graphite electrodes are used, Jain says the study does lend support to the idea that Joule’s law will continue to work for ionically conducting solids on the microscale.
“A reasonable agreement between the observed and calculated temperature values based on Joule’s law gives credibility to the applicability of this law at the microscale,” Jain says. “Notwithstanding, we are well aware of the simplifying assumptions of our calculations and other observations that indicate the presence of additional mechanisms of heating as well. So we need to conduct more detailed experiments and accurate modeling.”
Currently, Jain says the researchers are focused on exploiting their observations in practical applications, such as in the structuring of glass surface.
The open-access paper, published in Scientific Reports, is “Development of highly inhomogeneous temperature profile within electrically heated alkali silicate glasses” (DOI: 10.1038/s41598-019-39431-8).
Author
Lisa McDonald
CTT Categories
- Basic Science
- Glass