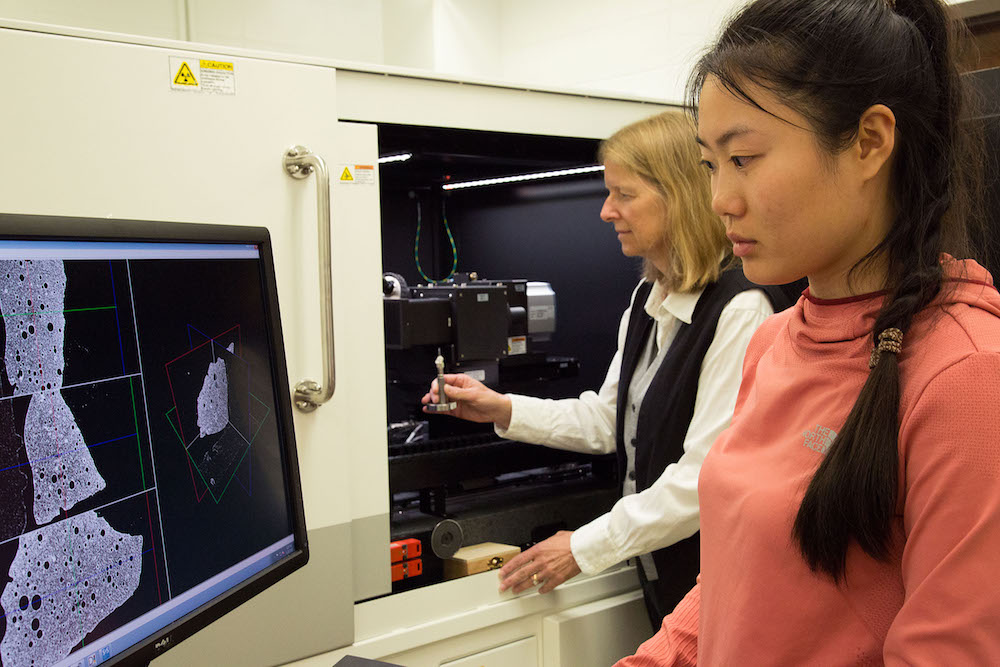
[Image above] Purdue University professor Laura Pyrak-Nolte (background) and physics graduate student Liyang Jiang (foreground) use a 3D X-ray microscope to examine additively manufactured gypsum rocks to better understand fracture formation. Credit: Purdue University
A topic that has caught my attention a lot recently is the idea of flow. Specifically, I find it interesting how numerous properties on the microscale, such as friction and roughness, greatly affect flow on the macroscale.
Understanding material flow is a big deal in many different fields, but it is particularly important in geology—understanding how the earth flows lets researchers predict and counter common natural disasters such as earthquakes and landslides. It also lets researchers engineer the earth for anthropogenic activities, such as long-term sequestration of waste and creation of hydrocarbon reservoirs and aquifers.
In these examples, flow of fluids through the earth is controlled by fracture geometry, i.e., the corrugated surfaces that emerge when a rock fractures. “Thus knowledge of the presence and orientation of corrugated surfaces enables design strategies for maximizing flow potential,” Purdue University researchers write in a recent open-access paper.
The Purdue researchers explain, though, that predicting how a rock will fracture is difficult.
“…the inherent heterogeneity in mineral phases and composition among rock samples causes a difficulty in identifying the contributions to surface roughness from each of these rock properties and processes, even when extracted from the same rock mass,” they write. Thus, “The spatial variability in compositional and structural features prevents reproducible measurements of fracture formation, deformation, and other physical and chemical properties.”
To ensure reproducible measurements of these various properties, the researchers took an interesting approach—they 3D-printed rocks.
“Through additive manufacturing, the orientation of the mineral fabric and layering can be controlled and used to determine the contributions to fracture surface roughness,” the researchers explain.
They additively manufactured gypsum rock by bonding layers of bassanite (calcium sulfate hemi-hydrate, 2CaSO4 ⋅ H2O) with a proprietary water-based binder. Then, they performed three-point bending tests to induce tensile fractures and used 3D X-ray computed microscopy to image the fracture propagation, followed by a laser profilometry scan to measure the fracture surface roughness.
The researchers discovered that when layers and minerals of the rock were oriented in the same direction, corrugated fractures tended to form. “The corrugations were enhanced in these samples because the layering and mineral fabric provided resistance to fracturing in the same direction,” they explain. In contrast, when the layers and mineral fabric were oriented orthogonally to each other, “corrugations in fracture surfaces are suppressed.”
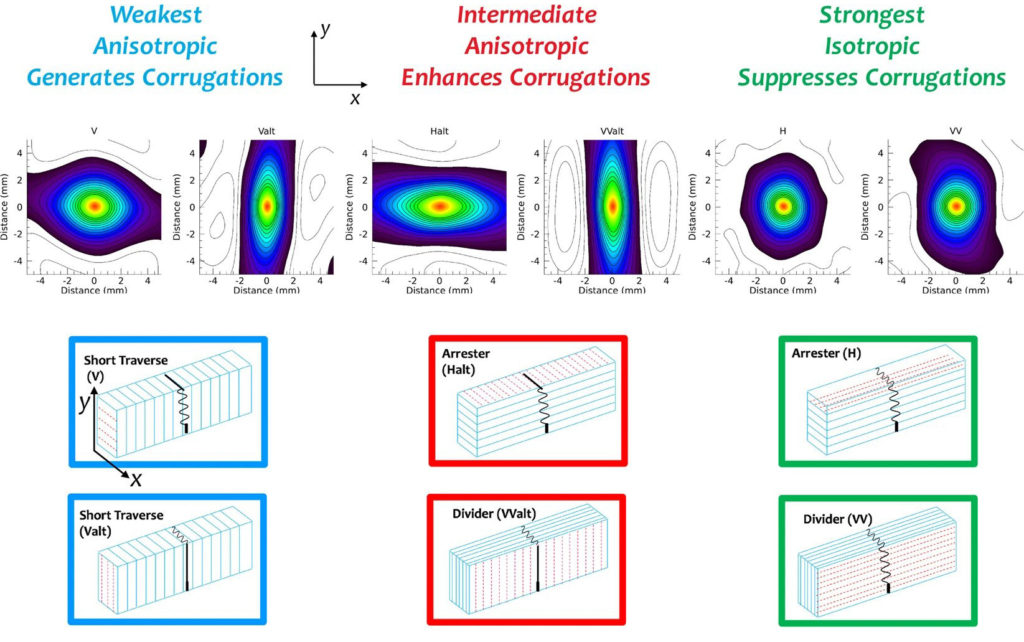
After the researchers observed this phenomenon, they tested randomly generated rock samples made with a traditional casting method, according to a Purdue University press release.
“They discovered that in rock samples with no layers and no oriented grains, fractures formed smoothly, with no corrugations,” the release states. “However, different roughnesses emerged in each sample because of the different mechanical qualities in the rock.”
These observations provided the researchers with clues about how the rock’s layers and grains affect the geometry of how it fractures, which is critical information for being able to predict fracture formation.
In the conclusion, the researchers note some limits of their study, such as the fact they only tested tensile failure. However, “The results presented here suggest that detailed mineralogical studies of cores should be performed to aid interpretation of preferred flow paths in existing fractures and to aid the design of induced fractures to maximize production potential,” they conclude.
The open-access paper, published in Scientific Reports, is “Mineral fabric as a hidden variable in fracture formation in layered media” (DOI: 10.1038/s41598-020-58793-y).