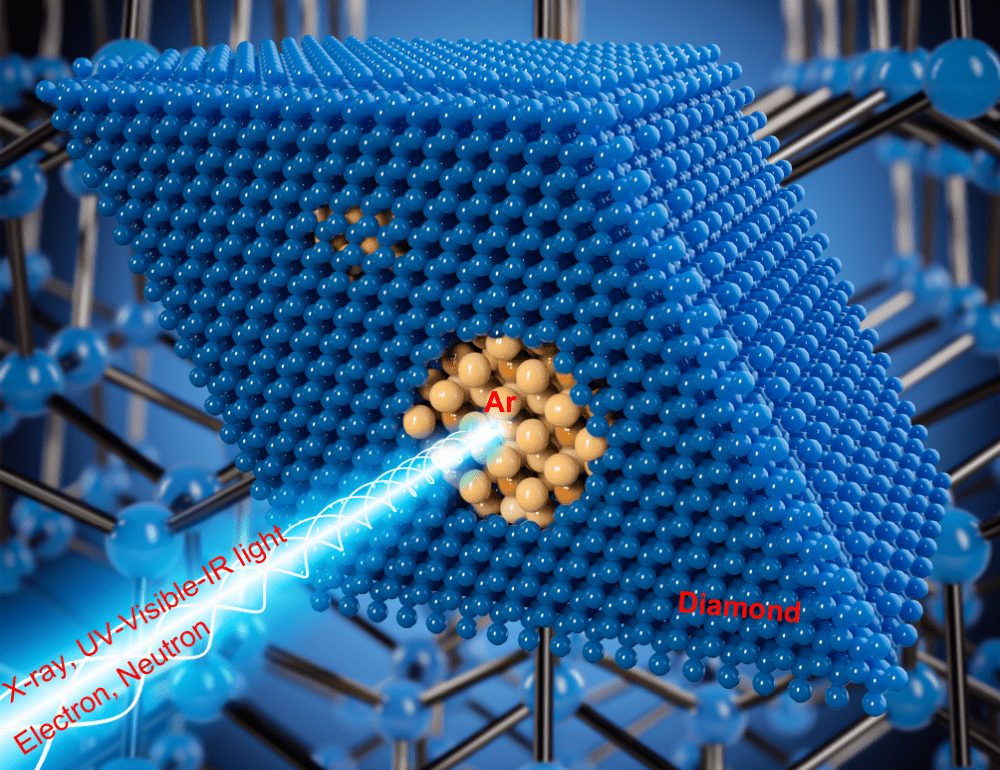
[Image above] Illustration of nanostructured diamond capsules with high-pressure argon nanodomains embedded in the matrix being analyzed with various characterization methods. Credit: Qiaoshi Zeng, Center for High Pressure Science and Technology Advanced Research
By Laurel Sheppard
Common material characterization methods such as electron microscopy and soft X-ray spectroscopy share the requirement that samples be placed in a near-vacuum environment.
For materials that only manifest their novel properties under high pressures, such as near-room-temperature superconducting hydrides and metallic hydrogen, this requirement excludes them from study with these techniques.
To overcome this barrier, scientists are searching for ways to retain a material’s high-pressure form once it is removed from a traditional thick-walled high-pressure vessel.
Previous research, such as here, demonstrated that carbon nanotubes can serve essentially as mini pressure vessels. Nonvolatile samples encapsulated in the nanotubes can be kept at tens of gigapascals of pressure, and because the walls are only nanometers thick, near-vacuum characterization methods can see through to the sample.
However, sealing materials and retaining high pressures inside carbon nanotubes is challenging, and this approach is not feasible for gas or liquid samples. A recent joint project by researchers in China and the United States showed that using diamond rather than carbon nanotubes to encapsulate samples may overcome these challenges.
The group is led by researchers from the Center for High Pressure Science and Technology Advanced Research in China and Stanford University in the U.S. The team also includes colleagues from the Shanghai Institute of Laser Plasma (China) and Argonne National Laboratory (U.S.).
Their process involves loading and sealing high-pressure samples within a capsule of ultrastrong, nanometer-thick layers of diamond. The diamond-encapsulated sample is then recovered at ambient conditions, preserving the high-pressure conditions of the sample as inclusions in the nanocrystalline diamonds.
Glassy carbon was chosen as the precursor for creating the diamond capsules because of its unique microstructure, which consists of numerous broken fullerene-like pieces containing nanoscale-enclosed, empty pores. The size and distribution of these pores are adjustable based on the polymeric precursor and pyrolysis temperature. Additionally, the pores are very stable, which is why researchers commonly use glassy carbon as a calibration standard material for small-angle X-ray scattering (SAXS) experiments.
In the recent study, high-pressure volatile samples were sealed within these pores, after which the glassy carbon was converted to nanocrystalline diamond at optimized pressures and temperatures. The preserved pressure in the final diamond was determined by the synthesis pressure.
A preliminary experiment using gaseous argon demonstrated the diamond capsules’ potential. Glassy carbon is impermeable to gases at ambient conditions but absorbs argon like a sponge at high pressures.
In situ high-pressure SAXS results confirmed argon accumulated in the nanopores following high-pressure compression. When glassy carbon samples were compressed without argon, a broad scattering peak appeared in the plotted SAXS data, indicating many empty pores in the sample. However, when the samples were compressed with argon, the broad scatting peak disappeared, indicating the density of argon and glassy carbon now were similar.
Synchrotron X-ray diffraction analysis also showed the argon remained in the capsules at high pressure (22 gigapascals), even after the release of external pressure. Energy-dispersive X‑ray spectroscopy mapping in TEM at high spatial resolution confirmed the existence of argon inclusions, with sizes of around several nanometers, nonuniformly embedded in the diamond matrix.
A similar experiment using high-pressure neon showed it could be encapsulated as well. However, it also made clear the need to account for sample-specific properties of diffusivity, chemical reactivity, and relative compressibility to optimize pressure–temperature conditions for sample loading and sealing.
“These experiments can provide comprehensive understanding of the composition, structure, and properties of materials under pressure that would otherwise be unattainable. Even variable-temperature, high-pressure TEM studies could be realized by heating [capsules] in a TEM column using a heating or cryogenic stage, which further opens up a broad pressure–temperature range for in situ investigations and interesting discoveries with standard low-pressure probes,” the researchers conclude.
In addition, “…[diamond capsule samples] are in principle cumulative, with the potential for unlimited, multiple syntheses, thus removing the limitation by which high-pressure phenomena exist in only a tiny sample inside a large pressure vessel,” they add.
Next steps for the researchers include exploring whether high-pressure solid samples can be encapsulated using this technique, by mixing carbon with sample nanoparticles and then converting the precursor carbon into diamond.
The paper, published in Nature, is “Preservation of high-pressure volatiles in nanostructured diamond capsules” (DOI: 10.1038/s41586-022-04955-z).