[Image above] Despite some differences, water and glass are quite alike in many ways. Credit: Beatrice Murch, Flickr (CC BY 2.0)
By S. K. Sundaram
When Bruce Lee uttered those three words back in 1971, it immortalized them to the present day. As a glass scientist listening to PJ Morton’s current rendition of those words—with a little help from Stevie Wonder and Nas—in the International Year of Glass (IYoG 2022), I would rather say, “Be like glass.” Let me explain.
Despite some differences (see sidebar), water and glass are quite alike in many ways. We have science to back that claim.
Water and glass have similar coordination geometries with tetrahedral symmetry (Figure 1). Their density decreases when cooled before a critical temperature. They flow more under pressure. They have analogous crystal structures, particularly when molten silica is crystallized.
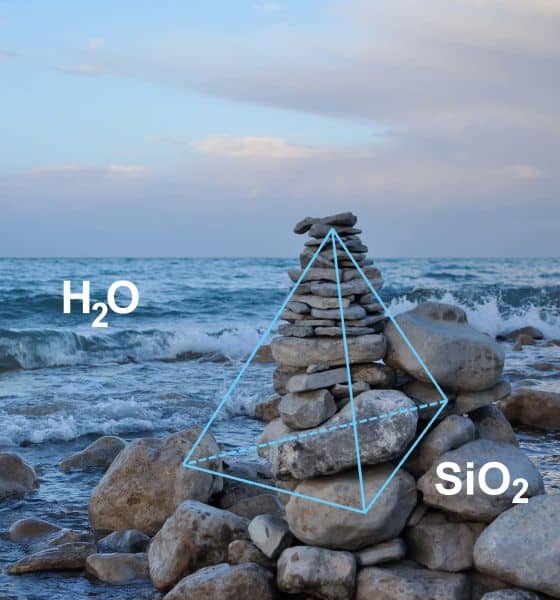
Figure 1. Tetrahedral symmetry of water (H2O) and silica glass (SiO2). Credit: Hajime Tanaka, The University of Tokyo
Differences between water and glass (silica)
Water is a liquid at room temperature, while glass (e.g., silica) remains a solid. Water freezes (crystallizes) at 0ºC to form ice. In contrast, molten silica does not easily crystallize. Instead, upon cooling, molten silica forms a disordered “glassy” structure.
During cooling, atoms in a liquid will slow down at a constant rate for a strong liquid. In the case of a fragile liquid, the rate increases rather monotonically. Water and silica do not follow these trends but show a maximum rate for the fragile–strong transition.
In a study published in PNAS, Professor Emeritus Hajime Tanaka’s group at The University of Tokyo used a two-state model of water and silica to explain their differing behaviors when cooled, which they further elaborated on in a follow-up paper.
They explain that both water and silica can be approximately treated as a dynamic mixture of two types of local structures (i.e., a two-state model). One is the disordered state (ρ) at high temperature, while the other is an ordered state (S) at low temperature. They used a structural parameter to describe the local structures thermodynamically.
Free energy change between the two states at temperature T and pressure P is ∆G = GS − Gρ= ∆E − T∆σ + P∆V, where ∆E, ∆σ, and ∆V are changes in the energy, entropy, and volume, respectively. The fraction of the S state (s), which is the controlling factor, is defined as s = 1/(1 + exp ((∆E − T∆σ + P∆V)/kBT), where kB is Boltzmann’s constant.
Changes in relative fraction of the ordered and disordered states based on temperature and pressure can explain several anomalous behaviors of water and glass, including glass and fragile–strong transitions.
Figure 2 below illustrates the two-state model, which reveals the striking similarity between water and glass, linking macroscopic behavior to microscopic states.
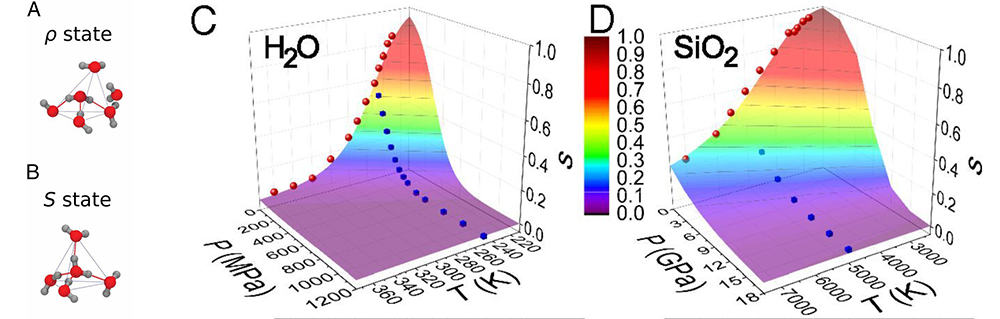
Figure 2. A and B: Examples of ρ and S states in water. The H-bonds are shown using red lines in a tetrahedral structure in blue. Credit: 2. Shi, Russo, and Tanaka, Proceedings of the National Academy of Sciences 115(38), 9444–9449, September 2018. C and D: s as functions of temperature and pressure. Datapoints in C and D are s values extracted from analysis. 3D surfaces show the two-state model fitted to s. Credit: Shi and Tanaka, Proceedings of the National Academy of Sciences 115(9), 1980–1985, February 2018.
In a press release, Tanaka says, “We showed that the macroscopic differences between water and silica originate in the microscopic world of bonding…We hope to extend this principle to other substances, such as liquid carbon and silicon, that are structurally similar to water and silica. The ultimate goal is to develop a general theory of how glass-formers differ from crystal-formers, which is something that has eluded scientists thus far.”
In summary, while water and glass have their differences, they both similarly continue to puzzle and defy scientists in many regards. That rebellious spirt is precisely what warrants the sentiment expressed in “Be like glass.”
The February 2018 paper, published in Proceedings of the National Academy of Sciences, is “Impact of local symmetry breaking on the physical properties of tetrahedral liquids” (DOI: 10.1073/pnas.1717233115).
The September 2018 paper, published in Proceedings of the National Academy of Sciences, is “Origin of the emergent fragile-to-strong transition in supercooled water” (DOI: 10.1073/pnas.1807821115).
Author
Lisa McDonald
CTT Categories
- Glass