[Image above] Credit: Nick Harris; Flickr CC BY-ND 2.0
[Updated 10/23/2015 to include SEM image from publication.]
Halloween is almost upon us, which means that pumpkins, ghosts, and skeletons are part of décor everywhere.
But who ever deemed skeletons as worthy of fright? I think our bones are more deserving of awe than eww or eek.
In a material sense, bone is fascinating—it’s porous, dynamic, and yet incredibly strong.
In a human sense, bone is fascinating—skeletons provide our bodies with the support to prevent us from looking like a Dalek’s innards. And yet skeletons are not simply frameworks—they’re alive. They grow, replace, remodel, and are anything but static, making repairing them incredibly tricky.
Current strategies to repair broken or damaged skeletal tissues often focus on providing the body with scaffolds to repair itself, rather than more historic approaches of trying to replace parts with stronger foreign materials. In fact, this sentiment was echoed by bioceramics experts at last summer’s Innovations in Biomedical Materials meeting. (Read my recap of the meeting here.)
Especially tricky is repairing joints—they combine bone with cartilage to provide structure yet flexibility and mobility. While modern repair strategies can mitigate damaged joints, they rarely achieve full functionality.
Part of the problem is that while we can develop man-made materials and spare parts that are stronger than the original, these foreign materials often have trouble integrating with the existing tissues.
Parts that are incompatible with the body’s immune system are rejected. And those that aren’t rejected often don’t fully fuse with the existing skeleton. Weak interfaces then lead to prolonged complications and issues with stability.
With that in mind, researchers at Tufts University (Medord, Mass.) and the University of Sydney (Australia) have developed a novel type of biodegradable scaffold that combines silk and ceramic to help broken bodies jointly rebuild the cartilage and bone that compose joints.
“It’s a challenging problem to tackle,” Rosemarie Hunziker, Director for the Program for Tissue Engineering at the U.S. National Institute for Biomedical Imaging and Bioengineering (NIBIB), says in an NIBIB news story about the research. “One of the big problems in cartilage tissue engineering is that the cartilage does not integrate well with host tissue after implantation, so the graft doesn’t ‘take.’ In this new approach there is a greater chance of success because the materials have architectures and physical properties that more closely resemble the native tissue.”
To match the two-part nature of joints’ osteochondral tissue—“osteo” meaning bone, and “chondral” meaning cartilage—the researchers developed a biphasic material that is flexible and strong, enabling joint movement while providing structural support.
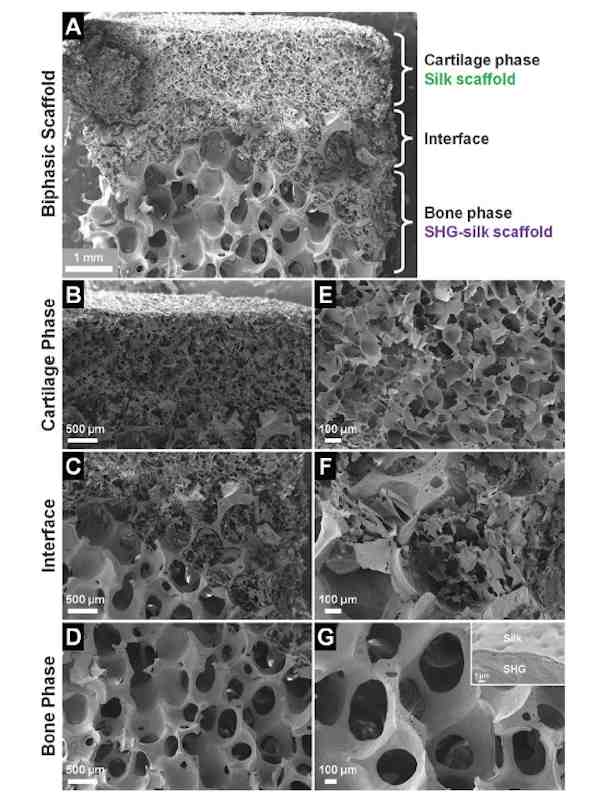
SEM image of the biphasic scaffold, showing (A) complete scaffold, (B) cartilage phase, (C) interface region, (D) bone phase, and (E–G) structural features at higher magnifications.
Reproduced from A biphasic scaffold based on silk and bioactive ceramic with stratified properties for osteochondral tissue regeneration with permission from The Royal Society of Chemistry.
“The goal was to develop an artificial scaffold with mechanical and bioactive properties that successfully promotes healing of damaged tissue to restore a fully functional joint. Bioactive properties include having a scaffold with the correct pore sizes that allow cells to enter and populate the scaffold after implantation, and being fully degradable over time to remove barriers to tissue regeneration” according to the NIBIB news story.
To create such a demanding bifunctional material, the team fabricated a cartilage-like scaffold from silk protein (from the silkworm Bombyx mori) joined to a bone-like ceramic scaffold of strontium–hardystonite–gahnite.
Researchers fashioned the ceramic material—fabricated from a mixture of Sr–Ca2ZnSi2O7 powder and aluminum oxide (Al2O3) powder (15 wt%)—into a scaffold via a polymer sponge method, in which they coated the ceramic slurry onto a sacrificial polyurethane foam.
Sintering the scaffold sacrificed the structural foam, leaving behind only the hardened ceramic scaffold.
This approach has found success with other teams recently, too. A news release from the University of Texas at San Antonio reported back in August that UTSA researchers are pursing similar strategies for bone repair. In the report, lead researcher Teja Guda says: “It almost looks like a kitchen sponge. The scaffold is 85% open space. The cells grow into it, and because we give them something solid to grow into, they start to regenerate tissue.”
The UTSA material has been successful in animal trials and “the product is now undergoing further development abroad,” according to the release. So there’s good reason to pursue scaffold materials to repair bone.
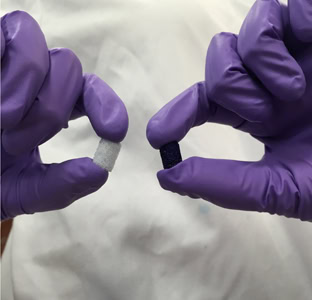
Pieces of the coated bone-mimicking foam biomedical engineering assistant professor Teja Guda has developed. Credit: University of Texas at San Antonio
The Tufts–Sydney team also coated its bone scaffold in silk protein solution “to facilitate adequate integration with the cartilage phase and allow control over size of the interface region, with the added benefit of enhancing the toughness of the ceramic scaffold by reducing the chance of crack propagation under load,” the paper states.
The researchers report that the biphasic scaffold maintained structural integrity during stretch and compression tests, standing up “under forces that were much higher than would be encountered in the body under physiological conditions.”
To test whether living cells regarded the new scaffolds as suitable homes to colonize, the team then performed in vitro experiments with cultured human mesenchymal stem cells.
Mesenchymal stem cells can differentiate, or develop, into a variety of other cell types, including cartilage and bone cells.
“The smaller pore size of the silk cartilage-like segment caused the mesenchymal cells to differentiate into cartilage cells,” NIBIB reports. “The larger pore size of the ceramic bone-like segment caused the mesenchymal cells to differentiate into bone cells.” And all without addition of bioactive molecules to the scaffold, a fact that would make potential commercial fabrication easier.
To confirm, the team validated that the differentiated bone cells expressed genes characteristic of bone cells, and that differentiated cartilage cells expressed genes characteristic of cartilage cells.
According to the report, the team continues to optimize its promising scaffolds for eventual in vivo testing in pigs.
The paper, published in Journal of Materials Chemistry B, is “A biphasic scaffold based on silk and bioactive ceramic with stratified properties for osteochondral tissue regeneration” (DOI: 10.1039/C5TB00353A).
Author
April Gocha
CTT Categories
- Biomaterials & Medical
- Material Innovations