[Image above] Leaving Halloween chocolate on your teeth too long can cause tooth decay—can we eventually repair teeth by growing new enamel? Credit: Steven Depolo, Flickr (CC BY 2.0)
We are now a (un)lucky 13 days away from Halloween and the beginning of the Día de los Muertos celebrations—and from spending $2.6 billion on candy around the nation.
Cheap candy is the staple (and bane) of Halloween handouts. And as much as you like eating it, the bacteria in your mouth like eating it even more, using it to produce an acidic byproduct that slowly eats away at tooth enamel.
Tooth decay is an extremely common problem around the globe. According to a 2016 global burden of disease study published in The Lancet, around 486 million children have decay in their milk teeth (baby, primary, or deciduous teeth), and about 2.4 billion people worldwide live with caries (dental cavities) in their permanent teeth.
In a perfect world, enamel would repair itself like other major body tissues, such as smooth muscle tissue. Unfortunately, because enamel is composed primarily of inorganic minerals, it does not naturally regenerate, which leaves dentists to repair it with alternative materials like metals, ceramics, and glass-ceramics.
If researchers could create enamel in the laboratory, it would be an ideal way to repair teeth. It would adhere well to existing enamel (unlike dental amalgam) and would match the existing enamel in hardness, thus avoiding unnaturally wearing down opposing teeth (as happens sometimes with ceramic crowns).
Creating enamel, though, is not simple. “Despite the great attempts made at enamel remineralization by using various strategies, such as direct solution mineralization, protein/peptide-induced mineralization, hydrogel-driven mineralization, and precursor assembly, no applicable repair for clinical development has been achieved because the complicated hierarchical structure of natural enamel cannot be replicated at large scale in laboratories,” researchers write in a recent paper.
The researchers come from Zhejiang University, Zhejiang University School of Medicine, and Xiamen University in China, and their paper published this August in Science Advances suggests a new strategy for growing enamel that builds off a previous attempt.
“There is growing evidence that biomineralization at the growth frontier occurs in an integrated crystalline-amorphous interface,” they explain in the paper. “Inspired by these biological processes, we suggest that a rationally designed structure between HAP [hydroxyapatite, Ca10(PO4)6(OH)2] and amorphous calcium phosphate [ACP; Ca3(PO4)2·nH2O] may mimic the biomineralization frontier to induce epitaxial regeneration of enamel.”
In a previous study using small ACP particles (~20 nm), the researchers found the particles could adsorb and even assemble onto enamel HAP crystals, but they failed to induce epitaxial growth of enamel crystals. “In general, coalescence and fusion between particles occur more readily at smaller sizes,” the researchers write. “Accordingly, a question arises: What is the minimum size of the ACP particles that can be used in HAP growth studies?”
To create smaller ACP particles, the researchers tested a different synthesis approach—instead of reacting Na2HPO4 solution with CaCl2 solution, they used calcium phosphate ion clusters (CPICs, ~1.5 nm) as the basic building blocks of ACP. (To prevent ultrasmall ion clusters from spontaneously aggregating, the researchers used organic compound triethylamine as a stabilizer.)
“In contrast to the other bulk ACP consolidated from nanoparticles, no particle, grain, or material boundaries could be observed within the CPIC-induced ACP by scanning electron microscopy (SEM) and atomic force microscopy (AFM), implying a structural continuity,” the researchers write in the paper. “This structural continuity can be extended to HAP-ACP interface to establish the mimetic biomineralization frontier.”
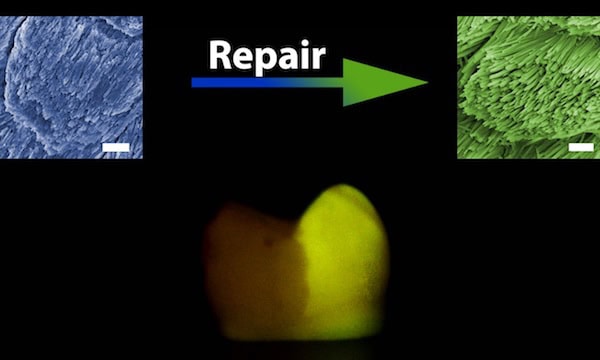
After their success inducing epitaxial regeneration with HAP (which serves as a simplified mineral model to investigate enamel formation and reconstruction), the researchers tried combining their CPIC-induced ACP with actual human enamel—with impressive results.
“In general, enamel rods run in a perpendicular direction to the enamel surface, and inter-rod enamel is at an angle of approximately 60° to the enamel rods … this unique feature of natural enamel could be precisely replicated within 48 hours by using the CPIC material,” the researchers write. “The boundary between the repaired and native enamel (protected by nail varnish during the repair) demonstrates successful epitaxial growth.”
While this early success is promising, there are still a few challenges to address before the method is viable for real-life use. In particular, the method repaired the enamel layer to about 2.5 μm of thickness; in application, it would need to be scaled up to 0.5–2 mm. Additionally, “there are a wide spectrum of dental cavities,” Shao Changyu, lead author of the study, says in a Zhejiang University press release. “We need to develop our regenerative model for different circumstances so as to ensure controllability and effectiveness.”
The paper, published in Science Advances, is “Repair of tooth enamel by a biomimetic mineralization frontier ensuring epitaxial growth” (DOI: 10.1126/sciadv.aaw9569).
Author
Lisa McDonald
CTT Categories
- Biomaterials & Medical
- Material Innovations
- Nanomaterials