[Image above] Credit: Paul Hocksenar; Flickr CC BY-NC-SA 2.0
When part of your bone is missing, messed up, or beyond repair, the question of how to replace it is a big one. Bone autografts—where a piece of bone is relocated from one healthy area of your body to the area of need—are the gold standard when it comes to replacing and implanting new bone. Bone can also come from a donor, living or deceased, which is called an allograft. But these procedures come with a risk of infection and rejection, especially in the case of allografts—not to mention that good bone is a bit hard to come by.
Synthetic bone substitutes sidestep some of these issues, but they often have other faults. While some subs, like NovaBone, are great for certain applications, structural stability in load-bearing areas is a concern.
“Using synthetic materials in this way is difficult and complicated because they need to be engineered to be porous and to replicate the various physical, chemical and mechanical properties found in natural bone tissue,” says Eddy Poinern, lead author of a new study from Murdoch University in Australia that investigated the biocompatibility of hydroxyapatite bone implants. “They also need to be non-toxic and have a degradation rate which will allow for cells from the host to steadily recolonize the area and permit the formation of blood vessels necessary for the delivery of nutrients to the forming bone tissues.”
The Murdoch team’s research, published in Scientific Reports, shows that hydroxyapatite pellets, sintered at just the right temperature to get a balance between porosity and strength, were porous enough to permit cell infiltration and stimulate new bone growth when implanted into the muscles of sheep.
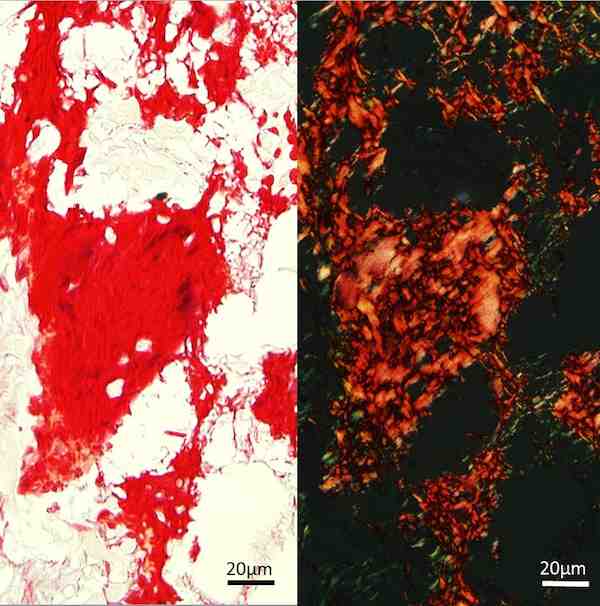
New bone matrix in the implanted pellets stains red with Picrosirius (left) and has a characteristic pattern under polarised light (right). Credit: Phil Nicholls; Murdoch University
The researchers sintered hydroxyapatite powder at temperatures between 650°C and 1250°C, based upon what they knew about how temperature affects grain growth and densification, to generate bioceramic pellets. Those pellets sintered at 650°C, 850°C, and 1050°C developed an airy porous structure, with porosity decreasing with increasing temperature.
To test biocompatibility, the scientists surgically implanted the pellets into sheep shoulder muscles. Not only were the pellets well tolerated biologically, their interaction with biological tissues exceeded expectations.
The work showed that, four weeks after implantation, the porous pellets were being infiltrated by a variety of types of cells, and, after 12 weeks, bone joined the mix migrating throughout the matrix.
“Our results were very positive—our pellets acted as a scaffold for the growth of bone material, made possible because of its porous properties allowing cells to infiltrate,” says Poinern.
What’s more, Poinern says, is that the pellets are economical to produce. The versatility of the material, combined with its promising performance so far, suggest it may be very useful in a variety of future medical applications.
“This material begins as a powder that can be theoretically moulded to any shape, or perhaps one day even 3D printed, then sintered to harden it,” says collaborating author Martin Cake, who implanted the pellets into sheep, in the release. The material could then be used to create custom implants or replacements depending on the patient and bone in need, an idea that is anything but new yet still largely unimplemented.
The paper is “The synthesis, characterisation and in vivo study of a bioceramic for potential tissue regeneration applications” (DOI: 10.1038/srep06235).
Author
April Gocha
CTT Categories
- Biomaterials & Medical
- Material Innovations