[Image above] A dying star, in orbit with a younger star, expels gas and dust within the Southern Ring planetary nebula (NGC 3132). University of Arizona researchers found evidence that complex carbon molecules could be forged in such environments. Credit: NASA’s James Webb Space Telescope, Flickr (CC BY 2.0)
In this year’s raw materials August issue of the Bulletin, which published online yesterday, we trace the materials supply chain to the very beginning—with formation of first glass in the early universe.
Author S. K. Sundaram, an Inamori Professor of Materials Science and Engineering at The New York State College of Ceramics at Alfred University, follows the timeline of universe expansion to determine when chemical elements, particularly oxygen and silicon, formed and interacted to form silica tetrahedra, the building block of silicate glasses and rock-forming minerals. “That will perhaps help us in defining the very moment the first glass was born,” he writes in the article.
Through his investigation, Sundaram makes it clear the universe contains many complex chemistries and phenomena yet to be discovered or explained. Fortunately, our ability to peer into the cosmos grows every year, and two recent studies offer a further glimpse into the vast unknowns of space.
Cloudy chemistry: Understanding the atmosphere necessary for silicate cloud formation
When you look into the sky here on Earth, the fluffy white clouds floating overhead consist mainly of water drops and ice crystals. But beyond our planet, clouds come in many chemical varieties. Clouds blanketing the top of Jupiter’s atmosphere are made of ammonia and ammonium hydrosulfide, for example, while Venus is surrounded by clouds composed largely of sulfuric acid.
Outside our solar system, telescopes have revealed clouds composed of silicates surrounding certain celestial bodies. Compared to the clouds mentioned above, silicates require much higher temperatures to undergo condensation. The exact conditions necessary for silicate cloud formation are detailed in a recent paper by two researchers at Western University in Ontario, Canada.
Stanimir Metchev, professor and Canada Research Chair at Western University, and postdoctoral scholar Genaro Suárez are the authors of the recent paper. In a NASA press release, Metchev says they did this study because the knowledge gained could help researchers better understand the atmosphere of a planet that’s closer in size and temperature to Earth.
In the paper, Metchev and Suárez used data gathered by NASA’s retired Spitzer Space Telescope on brown dwarfs, or substellar objects with a mass that falls in between planets and stars. Data from Spitzer suggests the presence of silicate clouds in a handful of brown dwarf atmospheres. However, in most cases, the evidence is too weak to stand on its own. So, Metchev and Suárez gathered more than 100 of these marginal detections and grouped them by the temperature of the brown dwarf.
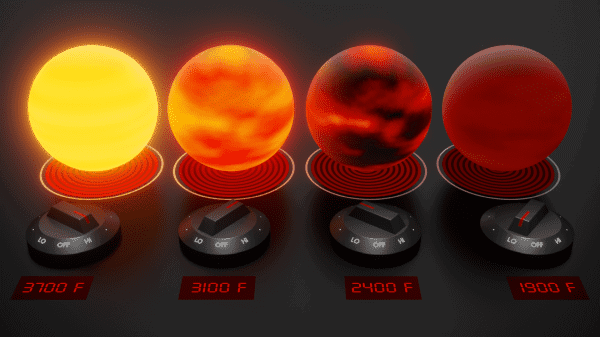
Silicate clouds may be visible in brown dwarf atmospheres but only when the temperature is between 1,900–3,100°F (~1,000–1,700°C). Too hot and the clouds vaporize; too cold and they turn into rain or sink lower in the atmosphere. Credit: NASA/JPL-Caltech
Their analysis revealed that all examples fell within the predicted temperature range for silicate cloud formation: between about 1,900°F (~1,000°C) and 3,100°F (~1,700°C). “We were very surprised at how strong the conclusion was once we had the right data to analyze,” Suárez says in the press release.
In a preprint version of their article, Metchev and Suárez say they hope these findings “will serve as a basis for planning and interpreting ultracool dwarf and exoplanet observations with the James Webb Space Telescope.”
The paper, published in Monthly Notices of the Royal Astronomical Society, is “Ultracool dwarfs observed with the Spitzer infrared spectrograph – II. Emergence and sedimentation of silicate clouds in L dwarfs, and analysis of the full M5–T9 field dwarf spectroscopic sample” (DOI: 10.1093/mnras/stac1205). Preprint version available at https://arxiv.org/abs/2205.00168.
Born from the ashes: Fullerenes and carbon nanotubes form from the dust and gas of dying stars
Among the allotropes of carbon, the fullerenes C60 and C70 hold a special place in space science. These ball-like molecules contain 60 and 70 carbon atoms, respectively, and are the largest molecules currently known to naturally occur in interstellar space.
Though we have known about the existence of C60 and C70 in circumstellar and interstellar environments for years thanks to data from NASA’s retired Spitzer Space Telescope, precisely how they form remains a matter of debate. A recent paper by researchers at the University of Arizona describes a mechanism by which fullerene formation may occur.
They start by noting that bottom-up formation processes are considered unlikely because timescales are too long to build such large molecules, given typical interstellar densities and temperatures. Thus, top-down synthesis seems to be more viable.
Recent laboratory experiments conducted by Bernal et al. (2019) showed that C60 can arise from rapid heating of silicon carbide grains with a cubic structure. Such grains are commonly produced in the circumstellar envelopes of stars nearing the end of their lives. As a star dies, there are numerous chances for the silicon carbide grains to experience rapid heating.
“[The grains] can be heated by shocks either in the late, thermal-pulsing stage … or in the proto-planetary nebula phase, where extreme mass loss occurs at high velocities. Also, stellar radiation may heat the grains as the white dwarf stage is approached,” they write.
To further explore this possible formation mechanism, the researchers conducted in situ heating experiments of silicon carbide grains using transmission electron microscopy. They used a different heating rate from Bernal et al. and without high-energy ion bombardment.
Their experiments revealed that heating the silicon carbide grains to the point of decomposition yields hemispherical C60-sized nanostructures that transform into multiwalled carbon nanotubes if held isothermally for more than 2 minutes.
The generation of carbon nanotubes is significant because, to date, researchers have not observed these carbon allotropes occurring naturally in space. The results support the likelihood that such materials can form in interstellar space.
In a University of Arizona press release, coauthor Tom Zega, professor in the UArizona Lunar and Planetary Lab, says that carbon-rich meteorites could possibly contain these complex carbon structures as well. Scientists will have the opportunity to explore this theory when samples from Bennu, a carbonaceous near-Earth asteroid that NASA’s UArizona-led OSIRIS-REx mission sampled, arrive in 2023.
The paper, published in Journal of Physical Chemistry A, is “Destructive processing of silicon carbide grains: Experimental insights into the formation of interstellar fullerenes and carbon nanotubes” (DOI: 10.1021/acs.jpca.2c01441).
Author
Lisa McDonald
CTT Categories
- Aeronautics & Space
- Basic Science