[Image above] Credit: whereareyousimon; Flickr CC BY 2.0
From infrastructure to automobiles to electricity, our manufacturing processes and the ways we consume energy are getting “greener.”
And the goal is clear: reduce carbon emissions.
The U.S. Environmental Protection Agency is developing stringent standards to reduce emissions, and this week India formally joined the Paris climate change agreement by officially adding the country to the list of United Nations members to ratify the plan.
But the challenge remains when it comes to transforming how we manufacture one of the most ubiquitous construction materials on the planet—cement.
Current cement manufacturing practices are carbon-intensive. In fact, today’s standard Portland cement production accounts for nearly 5% of the world’s total carbon emissions, so researchers are looking to nature for solutions to more sustainable concrete production.
In April, researchers from Rice University in Houston, Texas, took an atomic-level look at how concrete is produced. Rouzbeh Shahsavari, theoretical physicist at Rice, and his team published the results of computer modeling studies that reveal how dislocations—or screw-like defects—in raw crystals used for concrete affect manufacturing efficiency.
They found that tricalcium silicates (C3S) that consist of pure rhombohedral crystals are better than others for producing cement clinker—round lumps of C3S that, when ground into a powder, mix with water to make cement. When a clinker is easy to grind, manufacturers don’t need to work as hard, the team explained in a press release.
Beyond more efficient concrete manufacturing, Shahsavari and his team also wanted to find out what it takes to make concrete stronger and tougher. And after analyzing more than 600 computer models of concrete’s inner matrix, they published results in August that revealed both voids and portlandite particles are significant players in giving the material its remarkable qualities.
Fast-forward to this week, and Shahsavari and his team say they’ve delved deeper into concrete’s inner workings. The team has now detailed previously unexplored aspects that affect the energy required to make the widely-used material and the greenhouse gases released, according to a university press release.
After getting up close and personal with concrete at its most basic level, Shahsavari and his team developed techniques that allow them to analyze dislocations in belite, a dicalcium silicate component of Portland cement. As a result, they effectively detailed “how five distinct types of dicalcium silicates contribute to concrete’s ease of manufacture and ultimate strength,” the release explains.
“Though belite is crystalline in nature, the crystals are so small and the material so amorphous that nobody has looked at them with the kind of analytical eye they deserve,” Shahsavari says in the release.
But, Shahsavari explains, fine-tuning these crystals for use in the cement that holds concrete together can help save energy and ultimately reduce emissions.
“Putting an atomistic lens on the role of defects on the mechanics and water reactivity of belite crystals can provide new insights on how to modulate the grinding energy of cement clinkers and strength development of concrete,” Shahsavari explains. “Both of these factors can significantly contribute to energy saving and reduced environmental footprints due to the use and manufacture of concrete.”
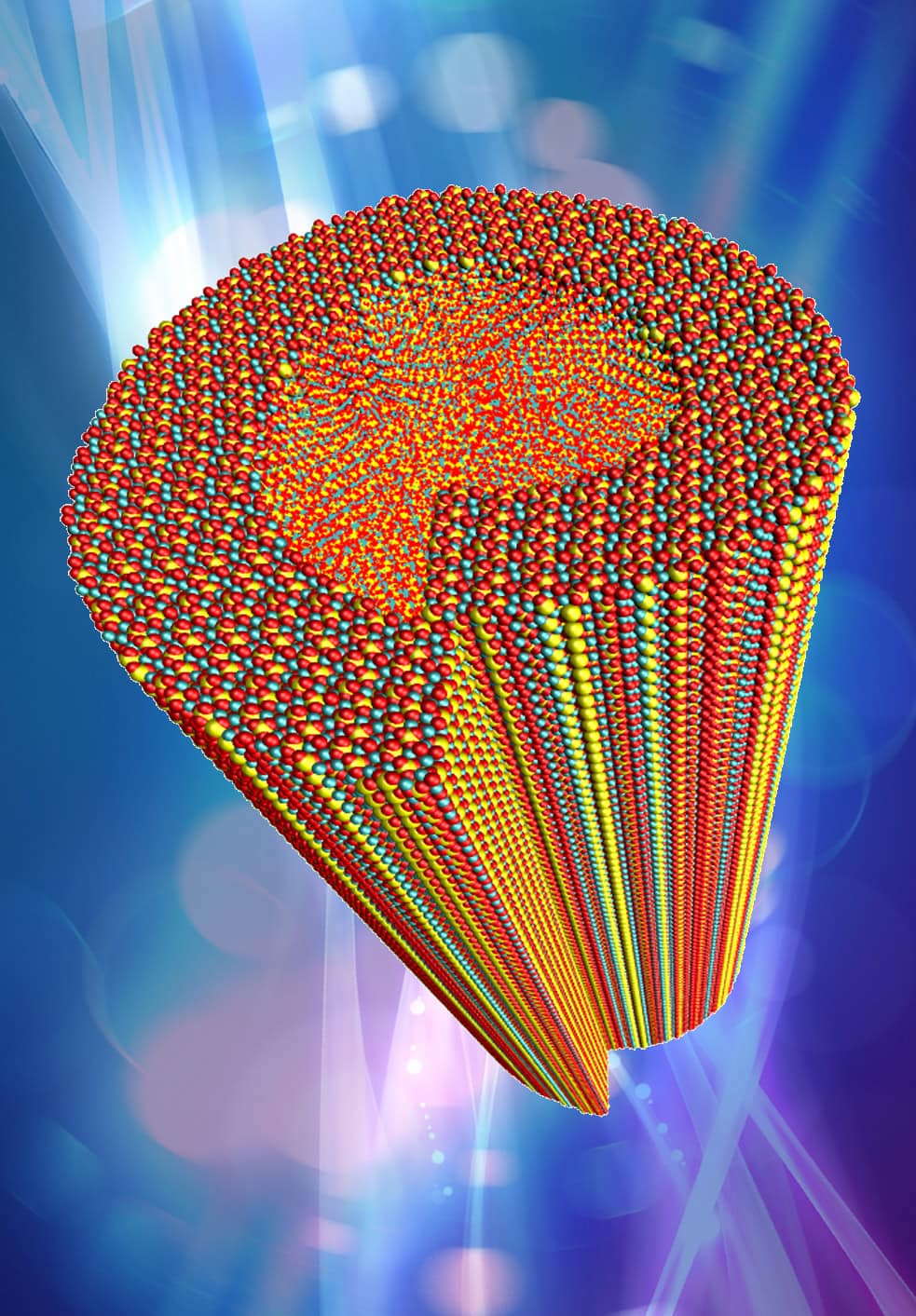
A close-up view of an edge dislocation defect in a cement crystal simulation. Credit: Lei Tao; Multiscale Materials Laboratory
Another advantage to using belite is that the crystals can be produced at a much lower temperature than tricalcium silicate, the more dominant ingredient in cement. “This temperature is at least 100°C (212°F) lower and makes belite an economical trade; however, it is harder to grind and reacts more slowly with water, which leads to delayed strength development in cement paste,” the release explains.
Shahsavari and his lab say their new approach to producing belite crystals could change that.
Belite crystals of calcium, silicon, and oxygen mainly take one of two different forms, the release explains—either monoclinic or orthorhombic—and each behaves differently at the atomic level.
The team subdivided these forms into five distinct polymorphic crystals, and, through computer simulations and high-resolution electron microscopy, “they determined one of the monoclinic forms—beta-C2S—is the most brittle and could be best-suited for cements requiring low-energy manufacture,” the release explains.
Shahsavari says this development could “put cement-based materials on equal footing with metallic systems and semiconductors in the emerging application of ‘defect-engineering’ to boost performance in manufacturability and functionality,” an innovation he expects “will lead to energy savings and environmental benefits.”
The research, published in Cement and Concrete Research, is “Edge dislocations in dicalcium silicates: Experimental observations and atomistic analysis” (DOI: 10.1016/j.cemconres.2016.09.012).
Author
Stephanie Liverani
CTT Categories
- Cement
- Construction
- Energy
- Manufacturing