[Image above] Schematic illustration of a design and fabrication process for porous bioceramic scaffolds. (A) Model design of the scaffolds. (B) Composition and preparation of the slurry. (C) Digital light processing printing process. (D) As-sintered scaffolds. Credit: Yin et al., Materials & Design (CC BY-NC-ND 4.0)
Chances are high you or a friend fractured a bone during the rambunctious years of childhood. Usually, these injuries healed after a few weeks in a doodle-filled cast. But in some cases, the size, shape, or placement of the break made the bone unable to heal on its own, thus requiring a bone graft.
Bone grafting is a surgical procedure that uses transplanted bone to repair and rebuild diseased or damaged bones. Traditionally, a surgeon would take bone from your hips, legs, or ribs to perform the graft. But in recent years, bioceramic scaffolds have attracted considerable attention as an alternative to natural bone due to its wider availability and bioactivity.
The structure as well as composition of a bioceramic scaffold plays an important role in its integration with the body. Fortunately, additive manufacturing techniques offer a way to fabricate personalized scaffolds with controllable and complicated features.
Digital light processing (DLP) is one of the most promising additive manufacturing technologies for preparing ceramic scaffolds with complicated fine features. DLP is a type of vat polymerization, in which a digital projector screen flashes an image across an entire resin layer, curing all points simultaneously. It is capable of developing features with fine resolution of less than 100 μm.
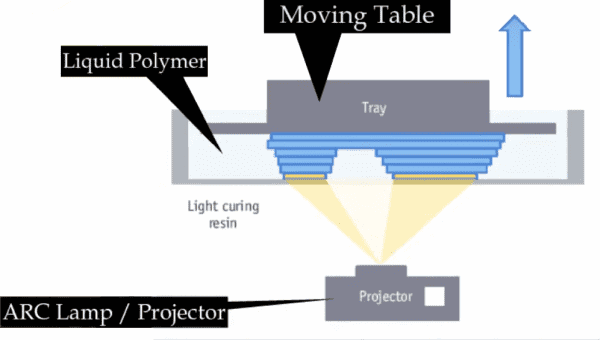
Illustration of how digital light processing works. Credit: Engineer’s Academy, YouTube
In today’s CTT, we look at three recent studies by several groups in China on advancing the use of DLP for fabricating bioceramic scaffolds.
3D-printed pink bioceramic scaffolds help treat tumor-induced bone defects
Compared to other types of bone defects, tumor-induced bone defects can contain residual tumor cells, which may lead to tumor recurrence after surgery. Traditional implanted bioceramics cannot treat such special tissue injuries, and so new bioceramics are needed that offer both tumor therapy and bone regeneration.
The bioceramic calcium titanate (CaTiO3) may offer both. Researchers recently showed that CaTiO3, when sintered at high temperature, will take on a pinkish color. This color change can broaden the ceramic’s ability to absorb electromagnetic radiation, giving it the ability to convert energy from near-infrared light into heat—which allows it to be used for tumor photothermal therapy.
Photothermal therapy is a minimally invasive and local treatment that induces cell death through extreme heat. The heat is generated by using electromagnetic radiation to heat special molecules called photothermal agents that are placed in the tumor tissue.
Compared to conventional photothermal agents, pink CaTiO3 scaffolds are naturally biocompatible and can aid in bone regeneration too. Thus, pink CaTiO3 can serve as a novel photothermal and bioactive biomaterial for tumor therapy.
In a recent study, researchers from Shanghai Institute of Ceramics, University of Chinese Academy of Sciences, and Nanjing Medical University used DLP to create pink CaTiO3 scaffolds for treating tumor-induced bone defects.
They note that usually it is not possible to create CaTiO3 scaffolds using DLP because CaTiO3 exhibits strong ultraviolet light absorption capacity, thus disrupting the ultraviolet crosslinking process. However, ultraviolet light absorption is diminished in sintered pink CaTiO3, which makes fabrication via DLP possible.
The researchers found that pink CaTiO3 scaffolds sintered at 1,400°C could kill tumor cells in vitro and inhibit tumor growth in vivo under near-infrared irradiation. Plus, pink CaTiO3 scaffolds sintered at 1,400°C and 1,500°C promoted the proliferation and differentiation of rabbit bone mesenchymal stem cells in vitro and presented osteogenic activity in vivo.
“Therefore, 3D-printed bifunctional CaTiO3 bioceramic scaffolds may have possible application in treating tumor-induced bone defects,” the researchers conclude.
The paper, published in Applied Materials Today, is “3D printing of pink bioceramic scaffolds for bone tumor tissue therapy” (DOI: 10.1016/j.apmt.2022.101443).
3D-printed bioceramic scaffold offers customized reconstruction of alveolar cleft
Cleft lip and cleft palate are among the most common birth defects. They are openings or splits in the upper lip and/or roof of the mouth (palate) that result from facial structures failing to close completely as the baby develops in the womb.
Of those who have a cleft lip or palate, about three-quarters also have an alveolar cleft, which is a gap in their upper alveolar bone (the thickened ridge of bone that contains the tooth sockets on the jaw bones).
Alveolar cleft is usually treated by grafting bone from the iliac crest (part of the pelvis) into the gap. However, many disadvantages are associated with autologous bone grafting, such as donor site morbidity, chronic pain, prolonged hospitalization, iatrogenic fractures, and risk of cutaneous nerve injury.
There are many studies on artificial biomaterials for treatment of alveolar cleft. The bioceramic tricalcium phosphate has garnered noticeable interest due to its good results in other bone repair studies. However, regarding alveolar repair specifically, tricalcium phosphate faces some challenges.
β-phase tricalcium phosphate scaffolds suffer from poor sintering ability and slow biodegradation rate, thus hampering its ability to repair the alveolar.
In contrast, α-phase tricalcium phosphate demonstrates excellent bone formation ability, but it suffers from premature absorption in vivo. To improve the use of α-tricalcium phosphate for alveolar repair, then, more research is needed on regulating its degradation time.
In a recent open-access study, researchers from Zhejiang University explored doping α-tricalcium phosphate with strontium to improve control over its degradation.
Previous studies showed that strontium can inhibit osteoclast activity, thus inhibiting bone resorption and cell-mediated biodegradation. So, “doping [strontium] in α-TCP [tricalcium phosphate] can theoretically slow down the degradation rate of α-TCP,” the researchers write. Plus, strontium can enhance osteoblast activity and cell differentiation and weaken inflammatory response, thus contributing to bone repair.
Testing showed the DLP-fabricated scaffolds consisting of 6% strontium-doped α-tricalcium phosphate demonstrated improved biodegradation rate, osteogenic capability, and prolonged mechanical stability in vivo compared to pure α-tricalcium phosphate scaffolds.
“This facile foreign ion doping in α-TCP and 3D printing route provides opportunities to develop the new-generation bioactive scaffolds for treating the challenging alveolar cleft repair and reconstruction,” the researchers conclude.
The open-access paper, published in Materials & Design, is “Customized reconstruction of alveolar cleft by high mechanically stable bioactive ceramic scaffolds fabricated by digital light processing” (DOI: 10.1016/j.matdes.2022.110659).
Gyroid-structured 3D-printed composite scaffolds allow for tunable degradation
As noted in the section above, controlling the degradation rate of a bioceramic scaffold is a key factor in its design. In a recent study by researchers at Huazhong University of Science and Technology, Wuhan University of Technology, and the Engineering Research Center of Ceramic Materials for Additive Manufacturing at the Ministry of Education, tuning degradability rate through material and structural design is explored.
Regarding material design, the researchers note that compared to hydroxyapatite and β-tricalcium phosphate, materials commonly used in bioceramic scaffolds, bioactive glass typically demonstrates superior biodegradability and bioactivity.
However, bioactive glass is a brittle material that is extremely sensitive to cracks. Thus, in the past few decades, many researchers have explored developing composite bioactive glass and ceramic scaffolds that exhibit both high bioactivity and good mechanical properties.
Regarding structural design, manufacturing bioceramic scaffolds with fine features using conventional forming methods is difficult to the brittle nature of ceramics. Fortunately, additive manufacturing technology, such as DLP, is making the fabrication of such fine-featured scaffolds much easier.
In this study, the researchers used DLP to fabricate gyroid-shaped ternary composite scaffolds composed of biphasic calcium phosphate (mixture of hydroxyapatite and β -tricalcium phosphate) and 45S5 bioactive glass.
Analysis showed the 45S5 bioactive glass can react with the biphasic calcium phosphate, thereby lowering decomposition temperature of the hydroxyapatite and preventing densification of the ceramic matrix. This process resulted in the formation of new major crystalline phases, Na3Ca6(PO4)5 and CaSiO3.
These phases helped enhance bioactivity and tailor degradability by exchanging ions such as Si4+, Ca2+, PO43−, and Na+ between the scaffold and simulated body fluid, resulting in the rapid formation of carbonated hydroxyapatite, which is analogous to the formation of natural bone tissue. Interestingly, the carbonated hydroxyapatite showed petal- and needle-like morphologies, which provided a large surface area to promote cell adhesion and proliferation.
Also, an increase in the bioactive glass content improved the scaffold’s degradability. Tunable degradability increased by three times at 30 wt.% bioactive glass and sharply increased by 6.8 times at 40 wt.% bioactive glass.
“This study provides a promising strategy to design scaffolds with improved bioactivity and tunable degradability to assist a diverse population suffering from orthopedic conditions,” the researchers conclude.
The paper, published in Biomaterials Advances, is “Three-dimensional printing of gyroid-structured composite bioceramic scaffolds with tunable degradability” (DOI: 10.1016/j.msec.2021.112595).
Author
Lisa McDonald
CTT Categories
- Biomaterials & Medical