[Image above] Researchers at Skolkovo Institute of Science and Technology (Skoltech) used function representation modeling and laser stereolithography to fabricate ceramic implants featuring complex internal pore structures. Featured is the design that showed highest compressive strength. Credit: Pavel Odinev, Skolkovo Institute of Science and Technology
In the latest December issue of the Bulletin, the cover story focuses on the use of ceramic and glass materials in the human body, from established products such as hip and knee replacements to newer applications such as bioactive glasses to repair soft tissues.
Additive manufacturing is one emerging technique in the biomedical field to produce ceramic implants. Additive manufacturing techniques are gaining traction in many fields due to their ability to fabricate products with detailed structures that are difficult or impossible to achieve through traditional manufacturing methods.
In the biomedical field, surface structure of implants and scaffolds is essential to successful osseointegration between the implant and the body. So it is no surprise that biomedical researchers are greatly interested in using additive manufacturing techniques to create implants and scaffolds with structures that improve osseointegration.
Porous bioceramics in particular may benefit from additive manufacturing. A porous implant features increased surface area, thus offering more opportunities for osteogenesis (bone formation) and vascularization (vessel formation).
Yet it is difficult to control the design of internal porous structures using traditional methods for creating porous bioceramics, such as foaming and burnt-out additives or replica templates. Additive manufacturing techniques, which construct a product from the ground-up based on a model, would in theory provide more control over the complex internal porous structure.
Preliminary work in this field using conventional computer-aided design (CAD) modeling to design implants runs into several difficulties. For one, traditional CAD models use polygons as the building blocks to construct models, which limits the variation of pores, strut thickness, and morphology of basic element intersections in the designed models. Additionally, the CAD model allows no easy changes in the grid-like implant topology, so tailoring the structure to a particular customer is cumbersome.
In contrast to CAD modeling, function representation (FRep) modeling overcomes these modeling limitations.
FRep modeling is based on geometry representation with mathematical functions. In other words, instead of constructing models using only polygons, FRep modeling also uses other mathematically described 3D objects like spheres, cubes, cylinders, and other shapes of greater complexity, thus opening the door to far more structure variations.
With just a few lines of code, these objects are iteratively combined through operations such as intersection, merging, and subtraction, resulting in complex geometric structures. Also, new parameters can be introduced into the equations and mathematical operators at every level of FRep modeling, so it is easy to tailor the structure for each customer.
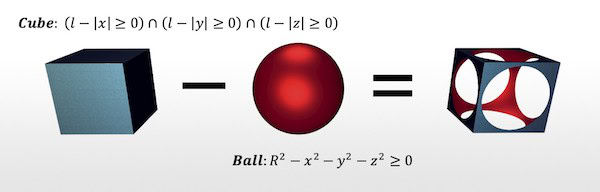
Example of operations used in FRep modeling to construct complex geometric structures. Credit: Skoltech Communications
FRep modeling is not a novel method. Yet “there are no publications on such application of this approach” for designing porous bioceramics, researchers explain in a recent open-access paper.
The researchers come from three context-driven meta-modeling research groups at the Skolkovo Institute of Science and Technology (Skoltech) Center for Design, Manufacturing and Materials (CDMM) in Russia. In the recent paper, they explore design and fabrication of porous ceramic implants using laser stereolithography directed by FRep modeling.
For this study, they focused on creating implants that could fill hole-like defects in trabecular bone, a light, porous type of bone that provides structural support and flexibility throughout the skeleton.
The ceramics additive manufacturing team of senior research scientist Svyatoslav Chugunov and engineer Andrey Tikhonov developed the overall concept and design of the cylindrical implants, which were 4 mm in diameter and 9.5 mm long. The team of professor Alexander Pasko, research scientist Evgenii Maltsev, and Ph.D. student Dmitry Popov prepared the FRep-based models, and the team of professor Alexander Safonov performed numerical validation of the implants’ mechanical performance using the finite element method (FEM).
Based on the numerical results, the researchers decided on four different types of structures for the implants, which differed based on the unit cells used to construct them, with varying thickness and length.
Eleven specimens of each type were manufactured using 3DMix alumina (Al2O3) paste as a ceramic feedstock in a Ceramaker 900 stereolithographic printer by 3DCeram (Limoges, France). The partner medical institution used ten specimens to test bone substitute materials in laboratory rabbits, and the researchers used the remaining specimens for mechanical testing.
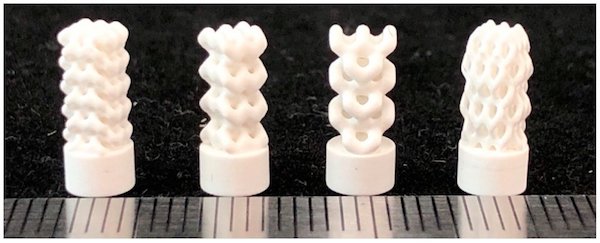
Photographs of the 3D-printed implants fabricated from models developed using FRep modeling. From left to right: Specimen BI001—the UC001 unit cell is replicated by a factor of 3 along the X and Y axes and a factor of 5 along the Z axis. Specimen BI002—the UC002 unit cell is replicated by a factor of 2 along the X and Y axes and by the factor of 4 along the Z axis. Specimen BI003—the UC003 unit cell is replicated by a factor of 1 along the X and Y axes and by a factor of 3 along the Z axis. Specimen BI004—the UC004 unit cell is replicated by a factor of 3 along all axes. Credit: Safonov et al., Applied Sciences (CC BY 4.0)
Mechanical testing showed that the BI001 specimen (the first specimen in the image above) is almost three times stronger compared to the other three specimens. In particular, its effective compressive strength (65.1 MPa) is comparable to actual trabecular bone (~50 MPa), which makes “it a promising bone substitute able to withstand high operating loads.”
The researchers attribute the higher strength of specimen BI001 to denser packing of the unit cells. Plus, “the BI001 specimen features three rows of contact surfaces in the upper part of the model, thus forming a larger area of contact with the loading plate and providing more uniform and less intense redistribution of load from the testing machine,” they add.
In future studies, the authors plan to conduct biomedical testing of the specimens, including cytotoxicity and osteogenic studies. They also intend to develop a novel method of ceramic additive manufacturing for porous structures to help reduce green body post-processing time and improve quality of the internal regions of the printed parts, according to a Skoltech press release.
The open-access paper, published in Applied Sciences, is “Design and fabrication of complex-shaped ceramic bone implants via 3D printing based on laser stereolithography” (DOI: 10.3390/app10207138).
Author
Lisa McDonald
CTT Categories
- Biomaterials & Medical
- Modeling & Simulation