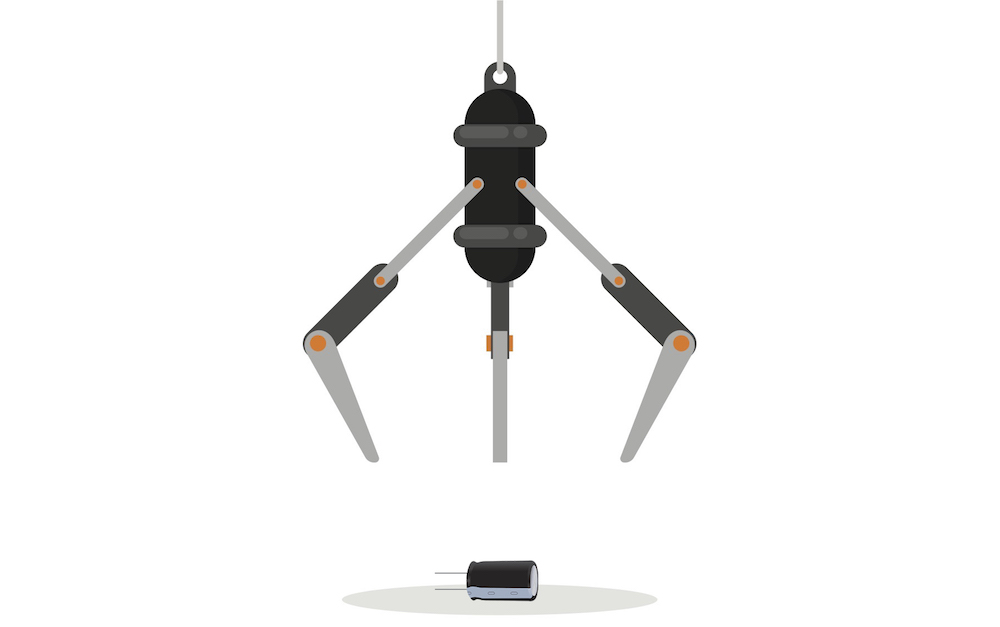
[Image above] Hafnium oxide-based ferroelectric capacitors could revolutionize nonvolatile memory devices—the goal now is learning more about these materials. Credit: ACerS
In the newly published January/February 2020 issue of the Bulletin, we celebrate an important anniversary taking place this year: the 100th anniversary of the first reported discovery of ferroelectricity.
Ferroelectricity is a property observed in certain materials characterized by the presence of spontaneous electric polarization that can be reversed in direction by applying an external electric field. University of Minnesota graduate student Joseph Valasek gave the first presentation on ferroelectricity in Rochelle salt at the 1920 Meeting of the American Physical Society. Since then, ferroelectric materials have become ubiquitous in electrical and electromechanical components and systems.
Our Bulletin feature article reviews the history of ferroelectricity, looks at its abundant applications, and briefly highlights some of the research areas scientists will focus on in the near future. One such area is ferroelectric random access memory (FeRAM).
FeRAM: An alternative nonvolatile memory device
Unlike many applications enabled by ferroelectric materials, FeRAM directly takes advantage of the switchable polarization ability of ferroelectric materials. (Most applications, such as pyroelectric sensors, use phenomena associated with this ability but do not directly leverage it.)
In FeRAM, a ferroelectric capacitor is coupled in series with a transistor. When the transistor is activated, the total amount of current (charge) flowing through the channel allows differentiation of the positive or negative polarization (memory) state of the ferroelectric capacitor. A voltage pulse is then used to set polarization of the ferroelectric for the next stored bit. The result is a nonvolatile memory device, i.e., a memory device that can store information even when its power source is turned off.
Compared to flash memory, another nonvolatile memory device and the most common type of storage for portable devices, FeRAM uses less power, demonstrates faster write performance, and exhibits a much greater maximum read/write endurance. Additionally, while flash devices have data retention times of about 10 years, some FeRAM products achieve 45-year retention.
Improving manufacturing compatibility: Hafnia to the rescue
Currently, FeRAM remains a relatively small part of the overall semiconductor market, in part because the materials used to make FeRAMs are not commonly used in complementary metal oxide semiconductor (CMOS) integrated circuit manufacturing. CMOS technology is the most common form of semiconductor device fabrication, and some of the materials used in FeRAM—specifically the lead zirconate titanate (PZT) ferroelectric layer and noble metals used for electrodes—raise CMOS process compatibility and contamination issues.
In 2011, Boscke et al. first reported ferroelectricity in fluorite-structured silicon-doped hafnia (HfO2) thin films. The observation of a switchable polarization in this material generated a great deal of excitement driven largely by the inherent silicon compatibility of hafnia, meaning hafnia-based FeRAM would be compatible in CMOS technology. Additionally, the fact that the first observation of ferroelectric response in hafnia occurred in films that were only 10-nm thick means hafnia-based ferroelectrics could enable scaling of existing ferroelectrics-based technologies to even smaller dimensions.
Quantifying electric potential in hafnia-based ferroelectrics
A lot of research is still needed to advance hafnia-based ferroelectric memory technology. Fortunately, researchers around the globe are willing to take up this challenge, such as the international team led by researchers at the Moscow Institute of Physics and Technology (MIPT).
MIPT researchers and their colleagues from the Deutsches Elektronen-Synchrotron (DESY) in Germany and North Carolina State University and University of Nebraska-Lincoln in the United States came up with a unique method for measuring electric potential distribution across a zirconium hafnium oxide (HZO) capacitor.
“An important role in the performance of … ferroelectric memory devices is played by the electrostatic potential, which dynamically changes in the process of memory operation,” the researchers write in the paper. “By proper engineering of the electrostatic potential profile across a ferroelectric HfO2 layer it is feasible to enhance the memory performance. To this end, it is critical to develop a methodology for the quantitative characterization of the electrostatic potential in the nanoscale ferroelectric devices.”
The method the researchers developed is based on hard X-ray photoelectron spectroscopy (HAXPES), a method that irradiates an excitation X-ray beam on the sample surface and measures the kinetic energy of emitted photoelectrons. The researchers previously used synchrotron-based HAXPES in a 2017 study to quantify polarization-dependent conduction band offsets at interfaces in ferroelectric TiN/HZO/TiN capacitors. In this study, they used near-total reflection (NTR) X-ray standing wave (SW) HAXPES to depth-selectively probe local electrostatic potential across the nanoscale ferroelectric HZO layer in functional TiN/HZO/W prototype memory capacitors.
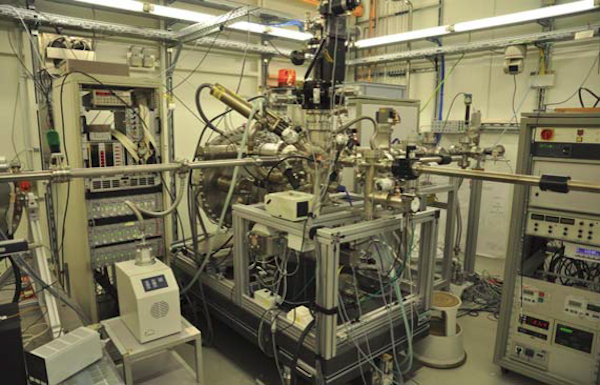
“The obtained potential profile across the ferroelectric HZO layer demonstrates that the depolarizing electric field is largely screened near the interfaces, and in the interior region of HZO, it nearly vanishes irrespective of polarization orientation,” the researchers write. “This observation indicates the presence of space charges in the interfacial regions of HZO, which effectively screen the depolarizing field.”
To identify what caused these space charges, the researchers performed depth-selective chemical and scanning transmission electron microscopy (STEM) analyses.
“The depth-selective chemical analysis of the TiN electrode pointed at the redox reaction at the top TiN/Hf0.5Zr0.5O2 interface yielding the formation of oxygen vacancies in the ferroelectric layer,” they explain. “The atomic-scale STEM analysis indicated the structural relaxation and local phase transition at the bottom Hf0.5Zr0.5O2/W interface presumably accompanied by the formation of defects with energy levels in the band gap.”
Ultimately, “Verified by our theoretical modelling, the proposed model explained the observed shape and changes of the electric potential in terms of polarization and depth dependent distribution of charged defects across the ferroelectric Hf0.5Zr0.5O2 layer,” they conclude.
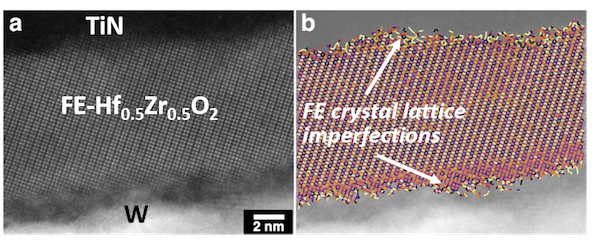
In an email, Andrei Zenkevich, coauthor of the study and head of the Laboratory of Functional Materials and Devices for Nanoelectronics at MIPT, says understanding what causes these defects is important because they can be detrimental to memory device operation.
“Indeed, non-ferroelectric charges in FE-HfO2 films … [can] cause the screening of the external electric field used to switch polarization/write information,” he explains. “These charged defects are believed to cause different effects in FE-HfO2 capacitor memory devices, such [as] ‘wake-up,’ ‘fatigue,’ and ‘imprint’ that affect their operation at different stages of the life cycle.”
“In order to eliminate these defects one needs first to find the way to ‘visualize’ them, and eventually to optimize the fabrication procedure,” he says.
In the future, Zenkevich says they plan to further investigate the effect of electrical cycling in situ on the device prototypes to see how it affects distribution of the potential (non-FE charges) across the ferroelectric layer and also to correlate with the fabrication procedure.
The paper, published in Nanoscale, is “Polarization-dependent electric potential distribution across nanoscale ferroelectric Hf0.5Zr0.5O2 in functional memory capacitors” (DOI: 10.1039/C9NR05904K).