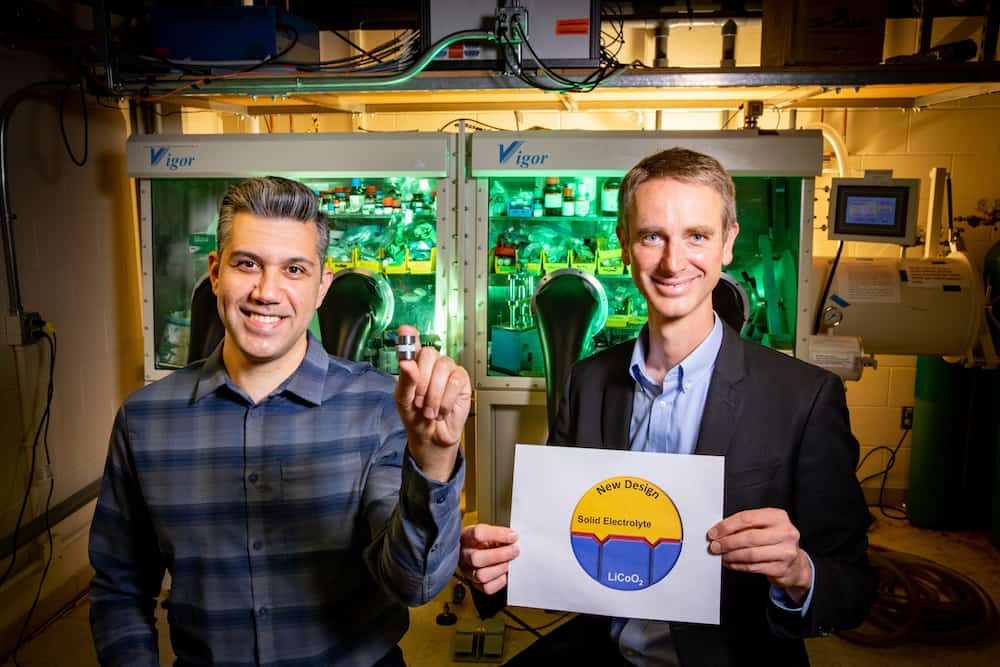
[Image above] Materials science and engineering researchers Beniamin Zahiri, left, and Paul Braun led a team that developed new battery electrodes made of strategically arranged materials in an effort to drive better solid-state battery technologies. Credit: Fred Zwicky, University of Illinois Urbana–Champaign
When it comes to the future of energy, solid-state batteries are one technology generating a lot of interest.
Solid-state batteries refer to batteries that contain a solid rather than liquid electrolyte, the substance that conducts ions and enables electric current to flow between a battery’s electrodes. Compared to conventional lithium-ion batteries, which use liquid electrolytes, solid-state batteries are expected to deliver higher energy densities and have less risk of catching fire.
Commercializing solid-state batteries, however, still faces some challenges. In particular, the interface between the solid electrolyte and electrodes is a vulnerable point for solid-state batteries.
“Currently, solid-state battery electrodes contain materials with a large diversity of surface atom arrangements. This leads to a seemingly infinite number of electrode-solid electrolyte contact interface possibilities, all with different levels of chemical reactivity,” Beniamin Zahiri, postdoctoral research associate at the University of Illinois Urbana–Champaign, explains in a UIUC press release. These reactions can affect interfacial stability, which can cause voltage loss and reduce power-generating ability, leading to poor battery performance.
To mitigate these interfacial reactions, many scientists have considered incorporating a thin coating material in between the electrodes and electrolyte to stabilize the interface, with some successes. However, coatings face some disadvantages.
“Coatings are usually made from poorly conductive oxide-based solid electrolytes, which pose two key disadvantages: a) decrease battery performance at high charge/discharge rates due to poor ionic conductivity of the coating, and b) impede any fundamental study of the impact of crystallography and morphology at the interface,” Zahiri says in an email.
In recent years, development of a new family of halide-based solid electrolytes has enabled the possibility of studying the interface without the need for coatings. And that is exactly what Zahiri and colleagues from UIUC and Xerion Advanced Battery Corporation (Kettering, Ohio) did in a recent paper, in which they investigated the role of interface crystallography and morphology on the performance of solid-state batteries.
In their study, the researchers used electrodeposition to create several dense, crystallographically oriented lithium cobalt oxide (LCO) and sodium cobalt oxide (NCO) cathodes. They paired the cathodes with four different solid electrolytes (three halide and one sulfide) so they could understand, “and ultimately exploit,” the role that interface morphology and crystallography play in solid-state battery performance.
Results from battery cycling tests showed “the considerable, unexpected and previously unobservable impact of crystallographic orientation, faceting and surface microstructure on [solid-state battery] performance,” they write in the paper.
Specifically, they found that the dense cathodes demonstrated a linear relationship between capacity fade and interfacial resistance—thus revealing a direct impact of interface crystallography and morphology on solid-state battery performance. In contrast, conventional composite cathodes with random crystallographic orientation and a larger interfacial area led to unpredictable behavior.
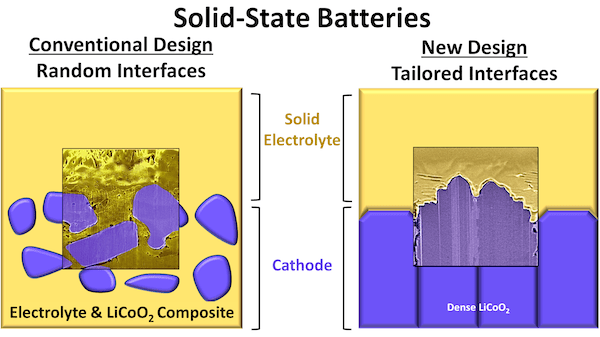
“[Interface atomic arrangement] is a new paradigm for how to evaluate all the important solid electrolytes available today,” John Cook, director of research and development at Xerion, says in the UIUC press release. “Before this, we were largely just guessing what electrode–solid electrolyte interface structures gave the best performance, but now we can test this and find the best combination of materials and atomic orientations.”
Zahiri says understanding interfacial instabilities and their root cause is just the beginning of exploring the underlying mechanisms of failure and improving the properties of interfaces through detailed thermodynamic and kinetic-based studies. “We plan to expand our studies to highlight these mechanisms that enable the entire solid-state battery community to discover new materials for next generation high-performance batteries,” he says.
The paper, published in Nature Materials, is “Revealing the role of the cathode–electrolyte interface on solid-state batteries” (DOI: 10.1038/s41563-021-01016-0).