[Image above] Alternative fuels like methanol are often created by burning fossil fuels. Effective artificial photosynthesis methods, however, would instead decrease atmospheric carbon dioxide by using the gas to produce alternative fuels. Credit: Hiram Cook, YouTube
Whether you are team solar, team wind, team hydro, or team geothermal, the fact stands that all of these renewable energy sources face the same drawback—none are actual fuels.
Each of these renewable energy resources allows us to generate electricity, but for us to efficiently and continuously use that electricity, we need methods by which to store it. And until new battery technologies come to fruition, relying entirely on electricity is not plausible at our current storage capability level—we still need fuel to generate energy.
But fuel does not need to come from fossils.
Hydrogen is an example of an alternative fuel that could be used in lieu of fossil fuels. According to the United States Department of Energy website, a hydrogen fuel cell coupled with an electric motor “is two to three times more efficient than an internal combustion engine running on gasoline.”
However, hydrogen and other alternative fuels like methanol exist in very small amounts in a natural pure form. Instead, these molecules reside within compounds that we need to break apart to extract the desired molecules. And unfortunately, the main method for extraction requires burning fossil fuels like natural gas and coal—meaning that creating alternative fuels produces carbon dioxide (the exact thing we are trying to avoid by using alternative fuels!).
However, an alternative way to create these fuels is artificial photosynthesis. Artificial photosynthesis produces hydrogen, methanol, and other organic molecules by using solar energy to break apart water and CO2. So, instead of producing CO2 like traditional methods, artificial photosynthesis decreases atmospheric CO2 by breaking it down to produce alternative fuels!
Currently, artificial photosynthesis is most successful at producing hydrogen because that only requires breaking down water. To create organic molecules like methanol, an additional step of CO2 photoelectrocatalytic reduction is required, which is a harder process to achieve. Additionally, the material commonly used to capture and catalyze water and CO2 from the atmosphere—titanium dioxide (TiO2) nanoparticles—is good at adsorbing water to its surface, but not so good with CO2. This means only one of the two raw materials required for artificial photosynthesis is effectively captured and brought into the process.
To increase performance of artificial photosynthesis techniques then requires two things: better adsorption of CO2 to the catalyst’s surface, and increased efficiency of the CO2 photoelectrocatalytic reduction process.
According to Andre Silva, a postdoctoral researcher at the University of São Paulo in Brazil, solving the first challenge can help solve the second.
“Higher CO2 adsorption increases the localized concentration of CO2 near the surface of TiO2, which increases the performance of the process,” he says in an email. Silva is the lead author on a new paper describing research he and his colleagues at the University of São Paulo, the University of California, Davis, and the Brazilian Nanotechnology National Laboratory performed on TiO2 nanocatalysts, research that looked to improve CO2 photoelectrocatalytic reduction during artificial photosynthesis.
In their study, the researchers looked to improve the reduction process both indirectly (increased CO2 adsorption capability) and directly (interface segregation) by doping TiO2 nanoparticles with barium oxide. Barium oxide was chosen as a possible dopant due to its CO2 adsorption capabilities, and its susceptibility to surface segregation (tendency to diffuse across the surface of the nanocrystals rather than the bulk).
“The interface segregation knowledge is one of the keys for designing the surface of any nanocatalyst,” says Silva. That is because segregation of ions is intrinsically connected to the thermodynamic stability of nanoparticles by the surface energy term. If a nanocatalyst is doped with the right ions, thermodynamic stability of the material will increase and surface energy will decrease, leading to “smaller crystallite sizes and higher specific surface area, which lead to more active sites for the reaction [to take] place,” explains Silva.
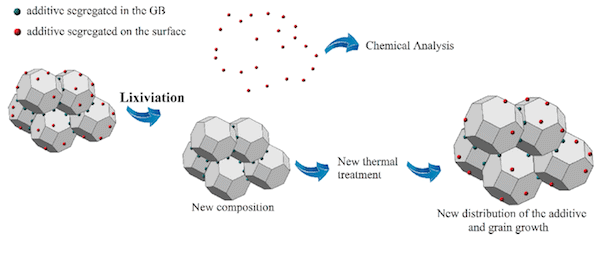
After doping with barium oxide and using a lixiviation method to quantify the barium ion content located at the surface and grain boundary interfaces, the researchers combined this knowledge with direct calorimetric measurements of surface energies and microstructural studies to reach a conclusion: surface segregation of barium ions improves nanocatalyst stability.
“This paper is a very good reference for understanding how to design nanoparticle surfaces to increase nanostability through surface segregation,” Silva says.
He adds that the study is part of a larger research program looking to decrease CO2 concentration using artificial photosynthesis: CO2 abatement programme—Project 31. The project is run by the Research Centre for Gas Innovation, a center supported by the São Paulo Research Foundation and Shell that studies the sustainable use of natural gas, biogas, and hydrogen, and management, transport, storage, and use of CO2.
“The next step of the research is to perform the artificial photosynthesis tests using a reactor that is being built in our group,” Silva says. “In the future, we believe that it will be possible to have portable systems, as panels with active nanoparticles that could be placed in many places, like houses or offices, to transform the CO2 from the atmosphere in useful organic composites.”
The paper, published in Journal of Physical Chemistry C, is “TiO2 surface engineering to improve nanostability: The role of interface segregation” (DOI: 10.1021/acs.jpcc.8b12160).
Author
Lisa McDonald
CTT Categories
- Energy
- Environment
- Nanomaterials