[Image above] Using liquid-phase and vapor-phase infiltration, researchers created glass-coated DNA origami scaffolds functionalized with metal and metal oxide particles. The superimposed graphics on this structure, which was imaged using a scanning electron microscope, reveal the layers of silica, alumina-doped zinc oxide, and platinum on top of the DNA origami scaffold. Scale bar: 1 μm. Credit: Michelson et al., Science Advances (CC BY-NC 4.0)
DNA, or deoxyribonucleic acid, is the molecule at the heart of genetic engineering. But what if it served as the basis for materials engineering as well? That is the idea behind the emerging field of DNA nanotechnology.
DNA nanotechnology is a materials design paradigm that uses DNA’s unique chemical and structural properties to create programmable nanostructures for applications both within and outside of biological contexts, such as digital data storage.
Of the various assembly techniques used in this field, DNA origami is a frontrunner. This technique uses numerous short “staple” strands of DNA to direct the folding of a long “scaffold” strand. The resulting scaffold has fixed dimensions and allows for the attachment of molecules at prescribed positions.
In July 2023, researchers at Columbia University, the University of Connecticut, and Brookhaven National Laboratory published an open-access paper that revealed a novel use for DNA origami scaffolds—as a framework for creating high-strength, lightweight glass nanolattices.
As explained in a September 2023 CTT, the researchers used sol-gel processing to coat the DNA origami scaffold in a thin layer of silica. The resulting glass-coated scaffold exhibited a yield strength higher than any known engineered materials with similar mass density.
The researchers noted that this nanolattice could serve as a stable platform for next-generation catalysts, battery cathodes, and other applications. However, for its potential to be fully exploited, they would need a way to incorporate other functionally active materials into the nanolattice.
To achieve this goal, several authors from the original study teamed up with researchers in the Soft and Bio Nanomaterials Group and the Electronic Nanomaterials Group at Brookhaven’s Center for Functional Nanomaterials. Through their combined expertise, the researchers successfully incorporated metal and metal oxide particles into the silica nanolattice, as described in a new open-access paper.
Their success relied on liquid-phase and vapor-phase infiltration, two emerging methods for converting polymer templates into functional organic–inorganic hybrids. The latter technique was pioneered by researchers in the Electronic Nanomaterials Group, who helped explore its potential to be used with the glass-based scaffold.
The researchers used the liquid-phase and vapor-phase infiltration methods both alone and together. They determined that combining these methods allowed for the creation of more complex structures.
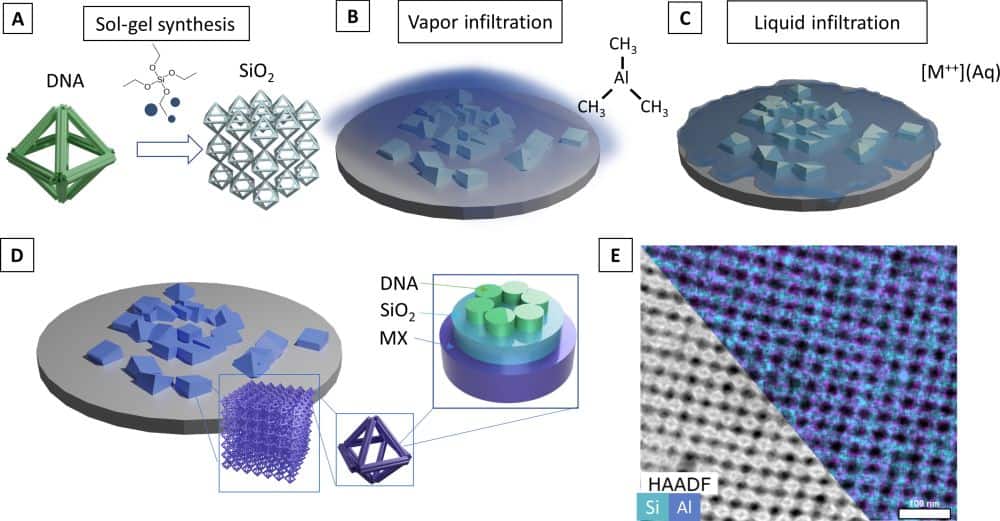
Schematic showing how (A) the glass-coated DNA origami scaffolds created through sol-gel synthesis were infiltrated with metal and metal oxide particles through either (B) vapor-phase infiltration or (C) liquid-phase infiltration, resulting in (D) a functionalized nanolattice after heat treatment. (E) Scanning transmission electron microscopy cross-sectional high-angle annular dark-field imaging and energy-dispersive spectroscopy map of silica (blue) coated with alumina (purple) via vapor-phase infiltration. Scale bar: 100 nm. Credit: Michelson et al., Science Advances (CC BY-NC 4.0)
Structural and spectroscopic analyses of the infiltrated nanolattices revealed a diverse set of nanostructures with varying electrical and optical properties. The underlying DNA origami scaffold remained preserved under the inorganic nanostructures.
In a Brookhaven press release, lead author Aaron Michelson, postdoctoral researcher at the Center for Functional Nanomaterials who began this research as a Columbia University graduate student, says it was surprising how many different material compositions of nanostructures they achieved using an identical process protocol.
“Typically for research like this, you need to spend a considerable amount of time with just one class of materials trying to get it to work, day in and day out. Whereas here, nearly everything we tried worked quickly, and at some point, we just had to stop producing structures because we wanted to write about it,” he says.
The structures described in the paper include various combinations of zinc, aluminum, copper, molybdenum, tungsten, indium, tin, and platinum, as well as composites such as aluminum-doped zinc oxide, indium tin oxide, and platinum/aluminum-doped zinc oxide.
With the potential of this new approach confirmed, the researchers plan to use the method in more complex research. They also plan to offer visiting scientists at the Center for Functional Nanomaterials access to the technique.
“We are always aiming to scale up our methods and connect new researchers to our developments. We want our work to benefit the wider scientific community, not just Brookhaven Lab,” says senior author Oleg Gang, materials scientist at the Center for Functional Nanomaterials and professor at Columbia University, in the press release.
The open-access paper, published in Science Advances, is “Three-dimensional nanoscale metal, metal oxide, and semiconductor frameworks through DNA-programmable assembly and templating” (DOI: 10.1126/sciadv.adl0604).
Author
Lisa McDonald
CTT Categories
- Basic Science
- Glass
- Material Innovations
- Nanomaterials