[Image] Grain boundary energy increases as grain boundary resistivity increases. Doping with a rare-earth ion, however, smooths defects and decreases energy, allowing for better conductivity at lower temperatures. Credit: Ricardo Castro, Reprinted with permission from The Journal of Physical Chemistry C, 2018, 122(46), pp. 26344-26352. Copyright 2018 American Chemical Society.
‘A chain is only as strong as its weakest link’
What is the first thing that comes to mind when you see this phrase? Maybe you think of the time you did a group project with that certain colleague who never pulls their weight. However, you may have taken the phrase in a more literal rather than figurative sense, in which case you would think of all the devices that break soon after purchase due to one component not pulling its weight (I’m looking at you, clip on mechanical pencils).
One technology that exemplifies this phrase is solid oxide electrochemical cells. Electrochemical cells are devices capable of either generating electrical energy from chemical reactions or using electrical energy to cause chemical reactions. Non-rechargeable batteries (AA, AAA, etc.) are a common example of an electrochemical cell.
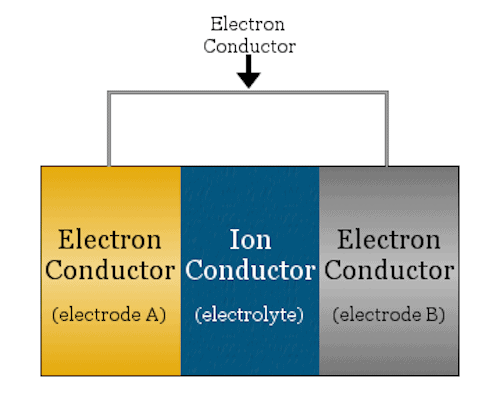
A basic electrochemical cell looks like a sandwich—the bread are the two electrodes (anode and cathode) and the meat is the ion conductor (electrolyte). Credit: Chemicool Dictionary
Solid oxide electrochemical cells are a specific type of electrochemical device that use solid oxide materials as the electrolyte, that is, the substance between the cathode and anode that allows ions to transfer between the two sides. And it is the solid oxide electrolyte that is the weakest link in striving for practical applications of solid oxide technology.
Solid oxide materials used as electrolytes in electrochemical devices require high operation temperatures. For example, cubic yttria stabilized zirconia—the most common electrolyte found in solid oxide fuel cells (SOFCs)—can only ensure reasonable performance at temperatures above 800°C (1,472°F). These high operation temperatures narrow the list of suitable materials for the remaining cell components—cathodes, anodes, and interconnects—and can lead to faster degradation of the device as a whole.
Compared to current fossil energy combustion technologies, SOFCs produce less CO2, consume less water, and use less fuel to provide the same amount of electricity. Additionally, unlike fuel cells that require pure hydrogen as fuel, SOFCs can produce electricity using existing gas transmission infrastructure, like natural gas and reformed diesel. Based on all these advantages, finding a way to lower the operating temperature range is a pressing research question—as evident by a new paper published in October.
Ricardo H. R. Castro, ACerS member and professor and associate dean for research and graduate studies at the University of California, Davis, and his colleagues from UC Davis, Idaho National Labs, University of Utah, and Russian Academy of Sciences, looked at how solid oxide electrolyte performance could be improved in a lower temperature range by mitigating the blocking effect of grain boundaries.
Grain boundaries are the interfaces between grains of differing crystal orientations in polycrystalline structures. An electrolyte containing many grain boundaries and/or defective grain boundaries cannot transfer ions as easily as an electrolyte with minimal interfaces. You can imagine grain boundaries like a landscape full of canyons. Crossing canyons takes energy, more so if the canyons are deep (i.e., grain boundaries are defective). If you (the ion) are to more easily cross canyons, either the canyons need to be less deep, or you need to remove the canyons entirely.
In their study, Castro and his colleagues looked to increase ionic conductivity in yttria-stabilized zirconia by pursuing the first option: smoothing the grain boundaries by doping with lanthanum. “There are the same number of [grain] boundaries,” Castro explains in an email, “but those [boundaries] have less defects (less broken bonds, for instance) and hence lower energy.”
Using scanning transmission electron microscopy-based energy dispersive spectroscopy, they observed segregation of lanthanum to grain boundaries (meaning lanthanum gathered along the grain boundaries and replaced the zirconium and yttrium atoms previously located there) and a reduction in local excess energy.
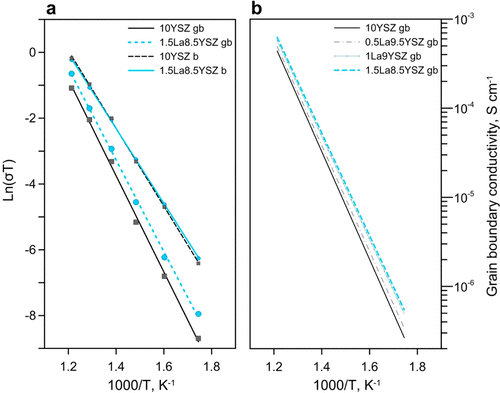
Bulk values of conductivity between samples were similar, but grain boundary conductivity values showed small incremental increases proportional to lanthanum concentration. (a) Ionic conductivity of bulk b and grain boundaries gb for 10YSZ and 1.5La8.5YSZ samples. (b) Ionic conductivity of grain boundaries gb for all samples. Credit: Ricardo Castro, Reprinted with permission from The Journal of Physical Chemistry C, 2018, 122(46), pp. 26344-26352. Copyright 2018 American Chemical Society.
When they then measured ionic conductivity using impedance spectroscopy in the range 300-550°C (572-1,022°F), they found that bulk values of conductivity between samples were similar, but grain boundary conductivity values demonstrated small incremental increases proportional to lanthanum concentration. For example, at 450°C (842°F), grain boundary conductivity values rose from approximately 3.96e-5 for the undoped sample (10YSZ) to about 5.22e-5 for the most-doped sample (1.5La8.5YSZ).
“The results suggest rare-earth ions with favorable grain boundary segregation enthalpy can smooth out the energy landscape across grain boundaries and thus facilitate ion mobility in the nanocrystalline electrolyte,” say the researchers in the paper.
As encouraging as these results are, Castro says it is only the first step of the research process.
“This was a proof of [concept] that the ‘healing’ of the blocking effect is possible by looking at the problem from an energetic viewpoint,” says Castro. “But identifying the best dopant is still an on-going research.”
The open access paper, published in The Journal of Physical Chemistry, is “A strategy to mitigate grain boundary blocking in nanocrystalline zirconia” (DOI: 10.1021/acs.jpcc.8b08877).
Author
Lisa McDonald
CTT Categories
- Energy