[Image above] New developments in materials science could enable the next generation of more efficient electronics. Credit: johngineer; Flickr CC BY-NC-ND 2.0
Are humans doomed?
I’m not referring to recent discussions about whether UFOs pose a national security threat (seriously, what are those things?!).
Instead, I think our end is more likely to be spelled out by our own doing.
Humans are decimating earth’s biodiversity. We have provoked more extreme weather patterns due to a warming climate. We are even the driving force for an entirely new epoch in planetary history. I mean, can earth possibly survive us? (Heyyyy, Mars…)
A couple of statistics really put this negligence and the scale of how wasteful we are in perspective. For one, we have never recycled 91% of discarded plastics on this planet, which will accumulate to an estimated 12 billion metric tons by 2050.
And second, an estimated 70% of all energy we produce is lost as heat. “It’s the biggest source of energy on the planet,” Joseph King, a program director with the Advanced Research Projects Agency–Energy (ARPA-E), says in a Yale Environment 360 article.
All that heat represents inefficiency in various processes, from large-scale energy factories down to individual electronic devices. But it all adds up to wasted resources and wasted energy, in some form or another—which contribute to questions of whether our existence on earth can continue to be viable.
Let’s put that existential crisis aside for a moment, shall we?
Instead, let’s focus on that more immediate problem of wasted heat because that is one area where materials science offers viable solutions.
For instance, when it comes to electronic devices, wasted heat is a big problem because it decreases efficiency of the devices, can damage their components, and ultimately reduces performance.
One strategy to address the problem of waste heat is to use materials, like gallium nitride, that offer less electrical resistance. Less electrical resistance translates to less friction and thus less waste heat that is generated in the first place.
An alternative strategy is to incorporate materials, like boron nitride, that can more efficiently dissipate waste heat within devices once that heat is generated. These strategies do not solve the problem of waste heat being generated, but instead mitigate its presence by getting rid of it more efficiently.
While such thermal management strategies do not solve the problem of waste heat, they can increase the efficiency and performance of electronic devices without completely overhauling their designs or making the switch to more expensive materials, such as gallium nitride. They can be quicker and cheaper fixes to the problem.
Realizing boron nitride’s potential
Boron nitride’s potential for thermal management in electronic devices stems from its predicted high thermal conductivity, a measure of its ability to transfer heat. That conductivity is critical for designing more efficient electronic devices because better thermal conductivity offers the ability to quickly dissipate waste heat.
Though boron nitride has long been predicted to have high thermal conductivity, actual experiments have failed to measure such high values. While hexagonal boron nitride’s thermal conductivity has been predicted to reach 550 W/m×K at room temperature, previous experimental results have only been able to demonstrate values of 220–420 W/m×K.
Now, researchers at the University of Bristol report that they have achieved even higher than predicted thermal conductivity by generating an ultrapure form of hexagonal boron nitride.
“Boron nitride is one such material which was predicted to have a thermal conductivity of 550 W/m×K, twice that of copper,” senior author and physics professor Martin Kuball says in a University of Bristol press release. “However, all measurements to date seemed to show its thermal conductivity was much lower. Excitingly, by making this material ‘ultra-pure’, we have been able to demonstrate for the first time its very high thermal conductivity potential.”
Natural variation in the boron isotopes (10B and 11B) in boron nitride creates inconsistencies in the material that reduce its thermal conductivity. So, the team controlled the composition of boron isotopes to fabricate a more pure form of the material.
Their measurements show that this isotopically pure version of boron nitride (monoisotopic 10B) has an in-plane thermal conductivity as high as 585 W/m×K at room temperature—even higher than the predicted 550 W/m×K.
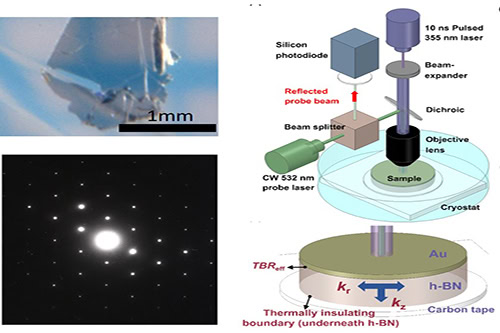
Ultrapure hexagonal boron nitride crystals (top left); area electron diffraction image showing a hexagonal diffraction pattern (bottom left); and schematic of the nano-second transient thermoreflectance technique (right top) and zoom-in on the carbon tape supporting the h-BN sample (bottom right). Credit: Yuan et al., Communications Physics (CC BY 4.0)
“The implications of this discovery are significant,” Kuball adds in the release. “Certainly, our reliance on electronics is only going to increase, along with our use of mobile phones and adoption of electric cars. Using more efficient materials, like boron nitride, to satisfy these demands will lead to better performance mobile phone communication networks, safer transportation and ultimately, fewer power stations.”
The open-access paper, published in Communications Physics, is “Modulating the thermal conductivity in hexagonal boron nitride via controlled boron isotope concentration” (DOI: 10.1038/s42005-019-0145-5).
Cozying up to polyaramide
But that is not all that is happening with boron nitride research of late.
Researchers at Rice University are also working with this promising material, and they recently devised a method to incorporate boron nitride nanosheets into a flexible nanocomposite material that has significant potential for electronics and more.
By combining 2D nanosheets of the ceramic material with 1D nanofibers of a flexible polymer, they created a thin, tough, and flexible high-temperature dielectric that satisfies demands of next-gen electronics that neither material can achieve alone.
“Ceramic is a very good dielectric, but it is mechanically brittle,” research scientist and first author M.M. Rahman says in a Rice news release. “On the other hand, polymer is a good dielectric with good mechanical properties, but its thermal tolerance is very low… When we combined the polymer nanofiber with boron nitride, we got a material that’s mechanically exceptional, and thermally and chemically very stable.”
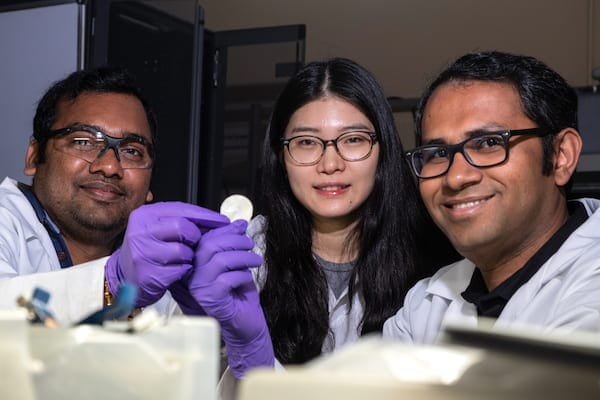
(From left) Postdoctoral researcher Anand Puthirath, academic visitor Fanshu Yuan, and research scientist M.M. Rahman show the high-temperature flexible dielectric material invented at Rice University. Credit: Jeff Fitlow; Rice University
To create the nanocomposite, the Rice scientists used a vacuum‐assisted layer‐by‐layer infiltration process to combine polyaramide nanofibers and boron nitride flakes (10% by weight). van der Waals interactions between the nanofibers and the flakes caused them to self-assemble together into thin nanocomposite layers, just 12–15 mm thick.
“The 1D polyaramid nanofiber has many interesting properties except thermal conductivity,” Rahman says in the release. “And boron nitride is a very interesting 2D material right now. They both have different independent properties, but when they are together, they make something very unique.”
Part of the uniqueness of the material is its ability to efficiently dissipate heat yet remain flexible, thanks to the contributions of the boron nitride flakes and polyaramide fibers.
The researchers say that in addition to having the ability to stack individual layers together to tailor the thickness of the nanocomposite, the process is scalable—meaning that integration into manufacturing processes is feasible.
The paper, published in Advanced Functional Materials, is “Fiber reinforced layered dielectric nanocomposite” (DOI: 10.1002/adfm.201900056).
Author
April Gocha
CTT Categories
- Electronics
- Material Innovations
- Nanomaterials
- Thermal management