[Image above] High-entropy oxide materials demonstrate superior thermal and mechanical properties needed for protecting turbine blades, such as those pictured above. Credit: Pro-Per Energy Services, Wikimedia (CC BY-SA 4.0)
As we pass through yet another record-hot summer in the northern hemisphere, it’s good to see that many corporations are taking seriously the need to reduce reliance on fossil fuels. Ford Motors, for example, recently announced their $11 billion investment in factories to produce electric-powered F-150 trucks and the batteries they will require. Because the F-150 is one of the most popular vehicles in the United States, this news is quite significant for getting a larger swath of American consumers out of fossil fuel transportation.
But it will be difficult, if not impossible, to convert all forms of transportation to electric drive systems. Certainly, transoceanic ships and aircraft will remain fossil fueled until such time as batteries achieve sufficient energy density for nonstop travel in places where electricity cannot be supplied. With this need in mind, efforts to develop more efficient engines proceed full-speed-ahead.
Increasing operating temperature is one way to improve the efficiency of turbine engines. As noted in many articles, including this CTT, ceramic matrix composites (CMC) with environmental barrier coatings (EBC) show great promise to meet the challenges of ultrahigh operating temperatures.
EBCs must protect the turbine’s core materials from chemical attack and thermal degradation while maintaining integrity under periodic and acute thermal stresses. A good EBC material has low thermal conductivity so that the temperature of the substrate remains low. Its coefficient of thermal expansion should be relatively high to match that of the substrate and connecting layers. The material should not react with silicates that are the major component of dust. Finally, the EBC should be crack resistant with high toughness and relatively low Young’s modulus.
Yttria stabilized zirconia (YSZ) with various levels of yttria are commonly used for EBCs. Their thermal expansion behavior is close to that of the substrates and their thermal conductivities are sufficiently low. However, they tend to have low mechanical damage tolerance and readily react with silicates. Thus, many researchers are working to uncover alternative materials with superior thermal and mechanical properties.
High-entropy oxides (HEOs) are among the classes of materials being explored for EBCs. As you may remember from your thermodynamics courses, entropy is a measure of the disorganization of structures, and the higher the entropy, the more stable the system.
In high-entropy oxides, disorder is achieved by using multiple cations of similar valence (charge) but dissimilar size. These cations randomly occupy sites in the crystal structure of the HEO. Given the right compositions, the different size cations distort the structure without disrupting it. This distortion leads to some very interesting properties, many of which are beneficial for EBCs.
Two recent articles in Journal of the American Ceramic Society explore different high-entropy oxide systems. Both studies use first principle modeling to determine the energetics of the compositions to map ranges of stability. This modeling compares the entropy of the proposed compositions and structures against unsubstituted constituent compounds.
In the first article, “High-entropy ferroelastic rare-earth tantalite ceramic: (Y0.2Ce0.2Sm0.2Gd0.2Dy0.2)TaO4,” the authors compare the rare-earth tantalite compound given in the title to the single rare-earth tantalites such as YTaO4 and CeTaO4, which all have monoclinic ABO4 structures. Four of the five rare-earth tantalites have the same space group, which is also shared by the mixed tantalite. However, Ce3+ is considerably larger than the other four rare-earth ions and has a slightly different atomic arrangement. With Ce3+ being placed into the “smaller” space group, it’s easy to envision the structural distortion.
The second article, “Multicomponent high-entropy Zr-Y-Yb-Ta-Nb-O oxides for next-generation thermal barrier coating applications,” explores various concentrations of the transition and rare-earth elements with ZrO2. The resulting materials have interspersed ZrO2 and ABO4 structures, ranging from monoclinic to single and double tetragonal phases. Interestingly, the Y3+ and Yb3+ ions are similarly sized and generally occupy the A sites, while much smaller but also similarly sized Ta5+ and Nb5+ ions occupy the B sites.
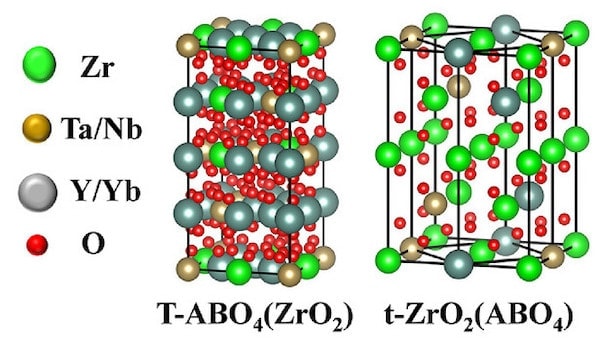
Models of the two tetragonal structures showing the interspersed ABO4 and ZrO2 arrangements. Credit: Yao et al., Journal of the American Ceramic Society
The disorder of these structures leads to thermal conductivities that are lower than those for the YSZ compositions used for comparison in each article. The authors of both explain that the distorted structure and different mass and volume of the ions lead to “phonon scattering” or deflection of the heat conduction. In other words, the thermal energy takes indirect pathways that are slower to transfer the energy than direct pathways.
The disorder also leads to higher toughness than for YSZs. The authors attribute this toughness to two factors. First, the distorted structures experience ferroelastic transformations under the stress of indentation testing. While the general arrangements of atoms are similar before and after the stress event, the ferroelastic transformation creates twins. Twinning is the rearrangement of the atoms to form a mirror image of the original structure, and the material absorbs the stress energy through this transformation. It is notable that YSZ also experiences ferroelastic transformations.
The second factor is deflection of cracks due to the stability of the high-entropy structures. Much like phonon scattering decreases thermal energy transmission, cracks formed by breaking of bonds cannot proceed directly through the grains. Instead, cracks are deflected to follow grain boundaries.
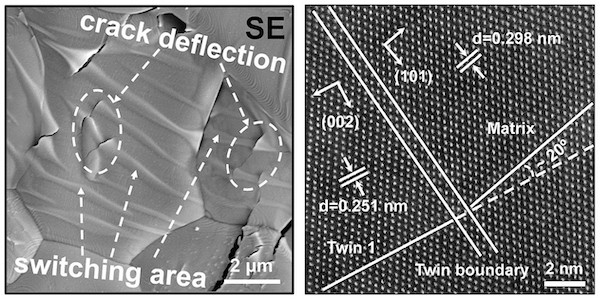
Images of crack deflection and twinning due to ferroelastic transformation. Credit: Yao et al., Journal of the American Ceramic Society
Unfortunately, many of the compositions tested by these authors exhibited lower thermal expansion than the YSZ materials they used for comparison. Fortunately, some showed expansion behaviors close to YSZ (and one even exceeded YSZ) over full or limited temperature ranges.
Finally, the two different papers diverged in the other properties tested. Most notably, the rare-earth tantalite was shown to have lower Young’s modulus and hardness than YSZ. The authors suggest these property values should make this material more damage tolerant than YSZ.
In the zirconia-based material study, the most promising composition was tested for silicate infiltration and showed superior results compared to the YSZ.
Overall, these studies demonstrate the potential of high-entropy oxide materials in high-temperature environmental coating applications. More studies are needed to move these materials toward commercialization, but fortunately researchers are actively pursuing this research. Just do a quick search for “high entropy” in ACerS journals and you’ll see there were 37 articles published on this topic in the past year!
Author
Jonathon Foreman
CTT Categories
- Aeronautics & Space
- Material Innovations
- Thermal management