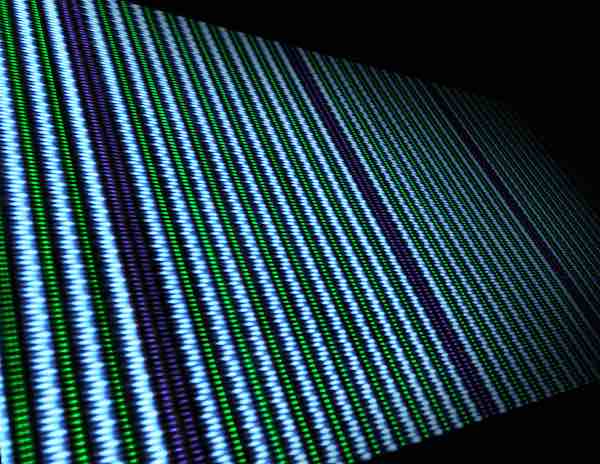
[Image above] A false-colored electron microscopy image shows alternating lutetium (yellow) and iron (blue) atomic planes. Credit: Emily Ryan and Megan Holtz; Cornell University
I vaguely remember a time in my early life when using a computer was an incredibly intermittent occasion.
Today, many of us don’t go more than a few minutes without using a computer, especially when you consider the processing power of some of today’s mobile devices.
And computers are still evolving—like many other facets of our connected modern lives, the continuing trend for electronics is to pack more power into a smaller device that requires less energy input.
While that’s a constantly moving target, new materials can make it happen. In fact, two significant materials research advances—one published in Nature and one published in Science—are moving precisely in that direction.
New magnetoelectric multiferroic material could mean faster computers that require much less energy.
Researchers at the University of Michigan have developed a new room-temperature magnetoelectric multiferroic material—one that could allow future computing devices to run on 100x less energy than current devices.
“Before this work, there was only one other room-temperature multiferroic whose magnetic properties could be controlled by electricity,” John Heron, assistant professor in materials science and engineering at the University of Michigan, who worked on the material with researchers at Cornell University, says in a Michigan news release. “That electrical control is what excites electronics makers, so this is a huge step forward.”
Room temperature multiferroics require much less energy to read and write data, and they also don’t lose data once they’re powered down—important benefits over today’s devices made from conventional semiconductors.
To create the new material, the researchers manipulated hexagonal lutetium iron oxide—a material consisting of repeating monolayers of lutetium oxide and iron oxide—which is robustly ferroelectric, but not particularly magnetic.
So, to increase the material’s magnetic properties, the team used molecular beam epitaxy to adjust lutetium iron oxide’s atomic arrangement, adding an extra layer of iron oxide every 10 monolayer repeats.
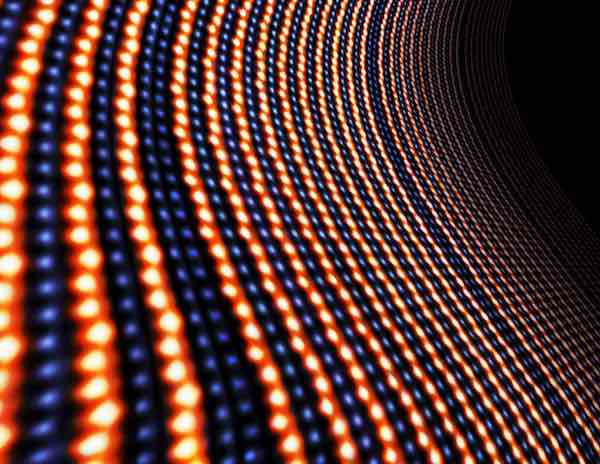
An extra plane of iron atoms was inserted every ten repeats, substantially changing the magnetic properties. The jagged appearance of the lutetium atoms is due to atomic rumpling. Credit: Emily Ryan and Megan Holtz; Cornell University
This atomic adjustment creates “planar rumpling” in the material, a phenomenon of atomic-level displacements that enhances the material’s magnetism. Applying an electric field to the material can adjust the position of the rumples, switching the magnetism of the neighboring layer of iron oxide and allowing storage of digital information, the release explains.
“We were essentially spray painting individual atoms of iron, lutetium, and oxygen to achieve a new atomic structure that exhibits stronger magnetic properties,” Darrell Schlom, ACerS member, professor of materials science and engineering at Cornell, and senior author of the new paper, says in the release.
And because electronics consume a significant portion of the world’s energy—an estimated 5% of total energy consumption, although that amount is project to increase by as much as 40%–50% by 2030—the research represents an initial step along the path toward significant energy savings in future electronics.
The paper, published in Nature, is “Atomically engineered ferroic layers yield a room-temperature magnetoelectric multiferroic” (DOI: 10.1038/nature19343).
Transition metal dichalcogenides could allow electronics to pack more power into still-smaller packages.
Integrated circuits are integral to modern electronics—which means that the circuits and the electronic components they contain are in a constant race to be able to pack more power into smaller packages. But at some point, the laws of physics must come into play. And transistor gates seem to be approaching their limits.
Transistor gates control current flow through transistors, meaning that they maintain the transistor’s ability to switch on and off with applied voltage—a critical characteristic for electronic devices.
Below a certain size threshold dictated by the laws of physics, however, electrons can ignore the gate’s stop or go commands through a process called quantum tunneling. Basically, the electrons can run amok.
At least that’s the case for transistors made of conventional semiconductors. So researchers at the University of California, Berkeley, Stanford University, Lawrence Berkeley National Lab, and University of Texas at Dallas have instead turned to new materials to build the tiniest transistor to date.
“The semiconductor industry has long assumed that any gate below 5 nanometers wouldn’t work, so anything below that was not even considered,” study lead author and graduate student Sujay Desai says in a Berkeley Lab news release. “This research shows that sub-5-nm gates should not be discounted. Industry has been squeezing every last bit of capability out of silicon. By changing the material from silicon to molybdenum disulfide, we can make a transistor with a gate that is just 1 nm in length, and operate it like a switch,” Desai says.
To bypass silicon’s theoretical limits, the team instead used the transition metal dichalcogenide to construct a tiny transistor channel. Because molybdenum disulfide’s electrons are heavier than silicon’s, they can be controlled with smaller gate lengths.
But building an even-tinier gate for a tiny molybdenum disulfide channel was tricky. Because conventional manufacturing techniques couldn’t craft something so small, the team instead used carbon nanotubes to build the gate.
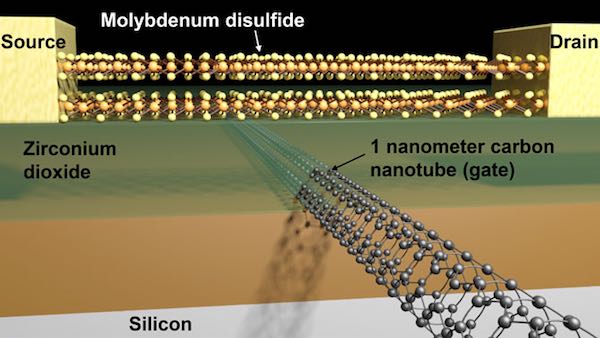
Schematic of a transistor with a molybdenum disulfide channel and 1-nm carbon nanotube gate. Credit: Sujay Desai; University of California Berkeley
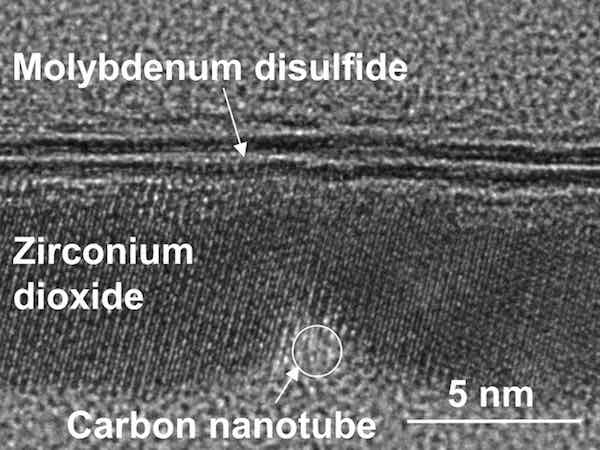
Transmission electron microscope image of a cross section of the transistor. It shows the 1-nm carbon nanotube gate and the molybdenum disulfide semiconductor separated by insulator zirconium dioxide. Credit: Qingxiao Wang; University of Texas Dallas
The team’s measurements showed that the combination of a molybdenum disulfide channel with a carbon nanotube gate worked—even at just 1 nm, the gate could control electron flow through the transistor.
“This work demonstrated the shortest transistor ever,” Ali Javey, lead principal investigator of the Electronic Materials program in Berkeley Lab’s Materials Science Division and UC Berkeley professor of electrical engineering and computer sciences, says in the Berkeley release. “However, it’s a proof of concept. We have not yet packed these transistors onto a chip, and we haven’t done this billions of times over. We also have not developed self-aligned fabrication schemes for reducing parasitic resistances in the device. But this work is important to show that we are no longer limited to a 5-nm gate for our transistors. Moore’s Law can continue a while longer by proper engineering of the semiconductor material and device architecture.”
The paper, published in Science, is “MoS2 transistors with 1-nanometer gate lengths” (DOI: 10.1126/science.aah4698).