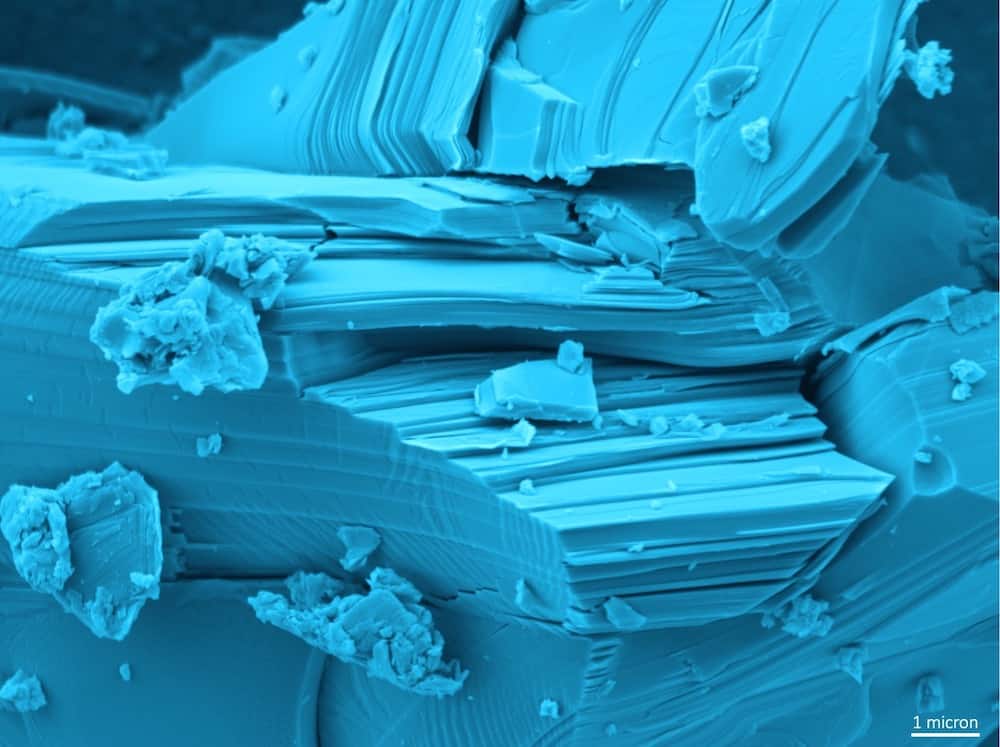
[Image above] Scanning electron microscope micrograph of a high-entropy MXene precursor, TiVCrMoAlC3. Credit: Kartik Nemani and Babak Anasori, Indiana University–Purdue University Indianapolis
Welcome back from the long 4th of July weekend!
While my holiday remained fairly low-key, I know many friends who went all-out with their celebrations to make up for the events canceled last year due to COVID-19. In some cases, my friends planned a bit too much and are starting this week exhausted. Yet packing your schedule is not necessarily a bad thing—sometimes adding more elements into a system can stabilize the performance, as is the case with high-entropy materials.
As explained in a previous CTT post, high-entropy materials consist of five or more elements of approximately equimolar composition. All the components in the material remain in a single homogeneous phase due to the system’s high amount of entropy.
High-entropy materials exhibit unique mechanical (strength, hardness, ductility, wear resistance), physical (magnetic, conductivity), and chemical (corrosion, oxidation, wear resistance) properties, which make them of interest for a wide variety of applications.
Despite the extensive number of studies on high-entropy 3D crystalline solids, very little has been reported on high-entropy 2D materials. “While a few studies explored high-entropy MPE [multi-principal-element] layered 2D transition metal dichalcogenides, to the best of our knowledge, there are currently no reports on high-entropy 2D carbides,” researchers write in a recent paper.
The researchers come from Indiana University–Purdue University Indianapolis (IUPUI), Argonne National Laboratory, University of Illinois, Chicago, and Norwegian University of Science and Technology. They are led by Babak Anasori, assistant professor of mechanical and energy engineering at IUPUI. In their study, they explored the possibility of creating high-entropy 2D materials using MXenes.
MXenes are a family of 2D transition metal carbides, nitrides, and carbonitrides originally discovered in 2011 at Drexel University. Last month, we covered a review article celebrating the 10th anniversary of this material family, which has shown itself useful in everything from optics and electronics to energy storage and harvesting to biomedical and environmental applications.
Anasori became involved in MXene research during his Ph.D. studies at Drexel University. He continued research on these materials during his postdoctoral time, and in 2015 he played an instrumental role in discovering a subfamily of MXenes called double-transition-metal MXenes, which contain two different transition metals in the MXene structure.
In an email, Anasori says the decision to explore creating high-entropy 2D materials using MXenes stemmed in part from the fact that “MXenes, right now, are outperforming other nanomaterials in many high-tech applications, due to their unique and tunable chemistry. We expect that these high-entropy nanoparticles will further enhance MXenes’ chemical tunability and increase their mechanical strength, stability, electrochemical and catalytic performance, and create novel electronic characteristics that are different from regular MXenes.”
The researchers first synthesized high-entropy layered MAX carbides TiVNbMoAlC3 and TiVCrMoAlC3 as the precursors for MXene synthesis. Through exfoliation and delamination, they obtained MXenes containing four transition metals—double the amount of transition metals used in traditional MXenes—and they verified the structure using X-ray diffraction, photoelectron spectroscopy, and electron microscopy.
The researchers carried out computational studies based on first-principles approaches to understand why this synthesis was possible, as well as to quantify the thermodynamic stability of the precursor MAX compositions.
They confirmed that maximizing configurational entropy in MXenes occurs in a fashion analogous to that in bulk disordered multicomponent systems. However, they added “further detailed studies on the nature of competing phases and formation pathways are required to understand the trends in synthesizability of high-entropy MAX phases.”
In the conclusion, they note that while this proof-of-concept study is an exciting first step, “the enormous compositional space coupled with the possibility of entropic stabilization in high-entropy MAX and MXene phases creates many different directions as well as challenges.”
Fortunately, the quickly advancing fields of artificial intelligence and machine learning are perfectly fitted for tackling this challenge, and the researchers suggest several such techniques that could be used in future studies.
In a IUPUI press release, IUPUI Ph.D. student and first author Kartik Nemani explains his excitement participating in this research.
“The idea of knowing that you know something that no one else on this planet knows about, and to be part of such fundamental research from the very beginning, is one of the best feelings a researcher can ever have,” he says. “And now with this expansion of the MXene family, the possibilities for how they can be used in the future [such as in quantum computing] have only grown.”
The paper, published in ACS Nano, is “High-entropy 2D carbide MXenes: TiVNbMoC3 and TiVCrMoC3” (DOI: 10.1021/acsnano.1c02775).