[Image above] Side view of a diamond anvil cell. The device was used to study conditions in which noble gas is incorporated into a diamond lattice during HPHT processes. Credit: Mark Stone, University of Washington
Be it Pinterest, Facebook, or Instagram, everywhere I turn the internet swarms with people showing off their minimalist Marie Kondo-inspired lifestyle. And in my age group, one place I see this minimalism pop up frequently is engagement rings.
Numerous articles of late point to the recent fad of favoring smaller diamonds over giant rocks (guess you can’t be a trendsetter in all things, Kim K). As many articles note, it is not the size but the cut of a diamond that really determines how beautiful—and sparkly—the ring will look.
“A well-cut diamond will bring that [brilliance and fire] out, no matter how big it is,” Jewelers of America spokesperson Amanda Gizzi explains in a Cosmopolitan article.
This statement is not simply hyperbole—take a look at nanodiamonds research.
Nanodiamonds are tiny diamonds that, by definition, are less than 1 μm (1,000 nm) in size, though most research focuses on nanodiamonds around 5 nm. Scientists first discovered nanodiamonds in the 1960s when using carbon-based trigger explosives like RDX and TNT to simulate nuclear explosions, and they discovered nanodiamonds in the residual soot.
While nanodiamonds have qualities in common with their larger siblings like hardness and inertness (and sparkle!), minimalists will not be running out to buy nanodiamond rings anytime soon—nanodiamonds are too small to be seen with the naked eye.
Even though nanodiamonds are not ideal for adorning rings, nanodiamonds work well as the centerpiece to numerous industrial applications, including as catalysts, seeding material, and additives for lubricants, galvanic coatings, and polishing compounds.
Though this list of applications is impressive, scientists hope to expand the benefits of nanodiamonds even further—by doping.
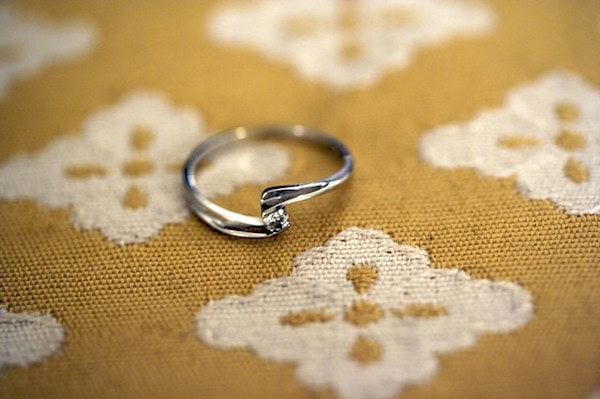
“The characterization and manipulation of dopants in diamond have generated a wide range of applications spanning quantum computing, sensing, and cryptography, biolabeling, determination of interstellar origin in meteoritic samples, and investigation of Earth’s mantle, due to the remarkable properties of the diamond host,” researchers write in a new open-access paper.
The researchers—from the University of Washington, United States Naval Research Laboratory, and Pacific Northwest National Laboratory—explain that the reason diamonds work so well as a host material is because the diamond lattice restricts heteroatom defect diffusion, meaning dopants are basically locked into place after they are inserted into the structure. This restriction enables scientists to reliably use single defects to complete tasks integral to quantum sensing, such as optically measuring local spatiotemporal variations.
However, due to diamond’s resistance to diffusion, doping by diffusion is not a practical option at ambient pressure and temperature. Instead, scientists typically have used ion implantation to dope diamonds, but this method can create extensive lattice damage and induce fragmentation of ions.
A bottom-up method for diamond synthesis—high-pressure, high-temperature (HPHT) synthesis—offers an alternative way to dope diamonds that poses less risk of damaging the structure than ion implantation.
In HPHT, a carbon-based starting material is placed under high temperature and pressure, turning the carbon into diamond. As previous studies have shown, if the starting carbon-based material is doped before HPHT treatment, then doped elements are incorporated into the diamond from the start rather than trying to insert elements into an already-formed diamond structure (as in ion implantation).
“In principle, it’s like making a cake: It is far simpler and more effective to add sugar to the batter, rather than trying to add sugar to the cake after baking,” public information officer James Urton notes in a University of Washington press release.
So, doping diamonds using an HPHT process is possible. But the researchers of the new open-access paper were curious if a specific type of doping is possible—doping with noble gas.
“[I]f incorporated into the lattice, [noble gases] have been proposed as defects for quantum sensing,” the researchers explain in the paper. “However, to date, noble gas defect formation, such as xenon-related dopants, has been restricted to ion implantation.”
“Despite its nearly ubiquitous role in high-pressure experiments, noble gas pressure media are widely considered to be inert, and there are no studies regarding the conditions that lead to incorporation within the diamond lattice under HPHT conditions,” they add.
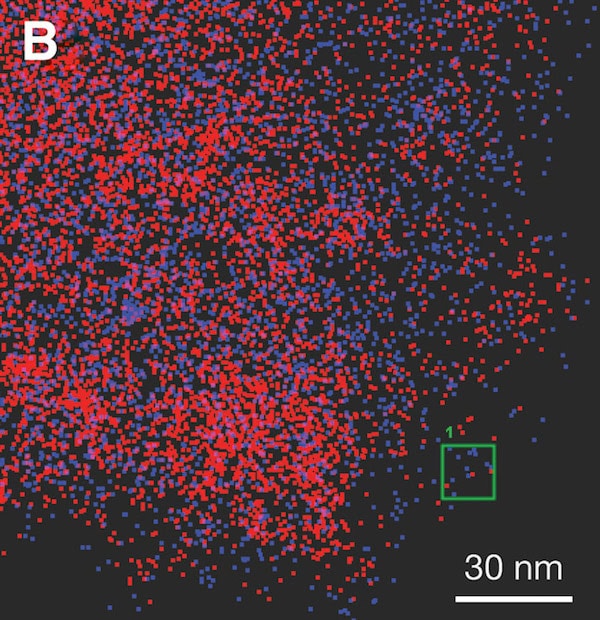
For their study, the researchers pursued two goals: 1) further investigate integrating a desired dopant into carbon before synthesis, and 2) investigate noble gas incorporation under HPHT conditions.
The researchers doped a carbon aerogel with tetraethyl orthosilicate (a silicon-containing molecule) and sealed the reactants within the gasket of a diamond anvil cell, using the noble gas argon as the pressure transmitting medium.
In regard to their first goal, the researchers found the silicon-based dopant molecules formed luminescent point defects in the diamond lattice, causing the nanodiamonds to emit a deep-red light at a wavelength of about 740 nm (perfect for medical imaging!).
In regard to their second goal, the researchers observed argon in all recovered diamonds synthesized at a range of pressures and temperatures, from 20–25 GPa and 2,780–4,940°F (1,527–2,729°C).
“While other reports have demonstrated the effect of noble gas pressure media on samples at elevated pressures … this is the first confirmation of noble gas doping during a HPHT phase transition and stable incorporation upon decompression to atmospheric conditions,” the researchers say.
Based on these results, the researchers emphasized two big takeaways in the conclusion of their paper. “For single-defect applications, this research opens the door to the incorporation of more complex defects into diamond with structures defined by the chemical dopant added into the carbon precursor,” they say. Additionally, “Given the prevalence of noble gas pressure media, these results have broad implications for high-pressure experiments, where, to date, noble gasses have been considered inert.”
The open-access paper, published in Science Advances, is “High-pressure, high-temperature molecular doping of nanodiamond” (DOI: 10.1126/sciadv.aau6073).
Author
Lisa McDonald
CTT Categories
- Nanomaterials