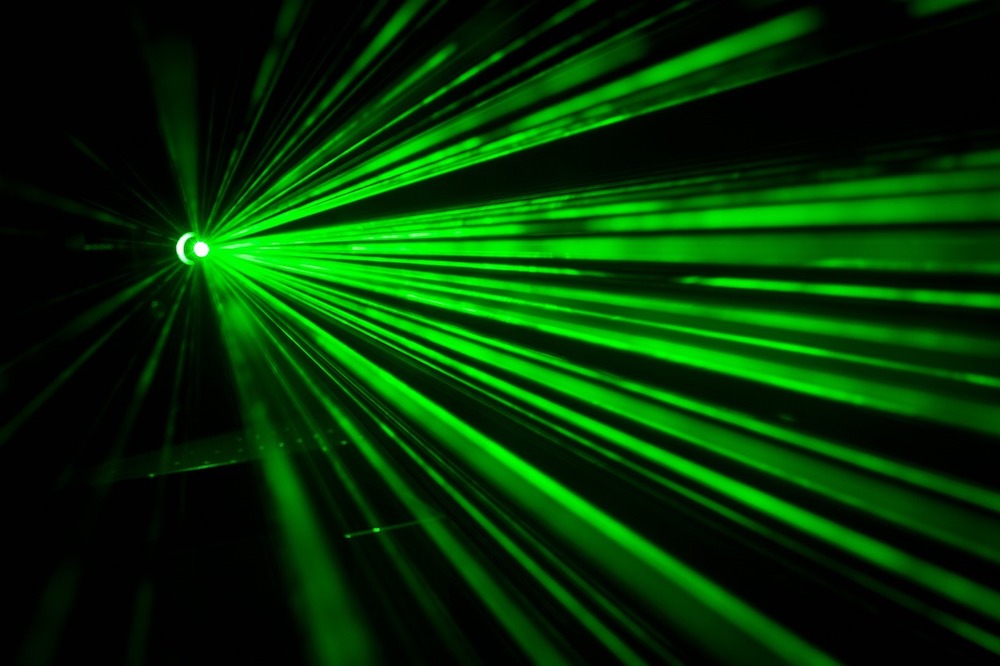
[Image above] Lasers are at the center of many advanced technologies. Developing new ceramic materials for use as the gain medium can improve a laser’s performance. Credit: Needpix.com
When I think of lasers, it is generally with amusement as I picture my cat chasing a red dot across the floor. But lasers are so much more than a cat toy—they are at the center of many advanced technologies, including information processing, imaging, and manufacturing.
In principle, lasers are fairly simple devices consisting of three main parts: an energy source, a gain medium, and an optical resonator. However, things get complicated when it comes to designing each of these three parts.
The gain medium in particular receives a lot of attention in laser research. It is the material used to amplify the power of light. Originally, single crystals were used for this purpose, but since the discovery of an optically transparent polycrystalline material with properties close to single crystals in the 1990s, so-called “transparent ceramics” now serve as the gain medium in many commercial lasers.
In recent years, researchers began expressing an interest in ceramic nanocomposites for use as infrared-transparent gain materials. Nanocomposites are multicomponent, multiphase solid materials in which at least one of the phases has at least one dimension on the order of nanometers. Compared to composites, nanocomposites tend to have higher thermal and mechanical stability.
Yttrium magnesium oxide ceramics (Y2O3–MgO) is one group of ceramic nanocomposites considered very promising for use as an infrared-transparent gain material. Both MgO and Y2O3 oxides weakly absorb in the range 0.3–6.0 μm and exhibit long wavelength cutoff, thus providing a wide transmission window. In addition, there is a stable bi-phase mixture that forms in a wide range of concentrations in the Y2O3–MgO system.
Maximizing the potential of nanocomposite Y2O3–MgO ceramics requires controlling the ceramic microstructure. Thus, many studies explore the influence of different variables on the microstructure, including manufacturing technique, fabrication parameters, and the addition of dopants. In today’s CTT, we discuss results from two papers published this year by an international team of researchers who explored some of these variables.
Maximize Y2O3–MgO potential: Sintering temperature and doping with holmium
The researchers come largely from Ukraine and Russia, though colleagues from China (first paper) and the Czech Republic and Romania (second paper) took part as well.
In the first paper, published in April, the researchers investigated the influence of sintering temperature on the structural and optical properties of 50:50 vol% Y2O3–MgO ceramics.
The ceramics were fabricated via spark plasma sintering (SPS) of nanopowders produced using the ecofriendly glycine-nitrate process. The researchers chose this fabrication method because of its perceived advantages, in particular the wide range of opportunities to vary sintering parameters with SPS and the ability to obtain fine particles with high purity in a short time through glycine-nitrate reactions.
They determined that maximum Vickers hardness of 10.9 GPa is achieved for ceramics sintered at 1,200°C; subsequent microhardness reduction between 1,250–1,300°C is caused by grain growth with decreasing creep limit. Infrared transmittance tended to increase with the sintering temperature due to increase of density and grain growth and decrease of interphase grain boundary extension. As such, Y2O3–MgO ceramic samples sintered at 1,300°С exhibited the highest infrared-transmittance of 71% at 6-μm wavelength.
“These results indicate that the mechanical properties and IR-transmittance can be improved by engineering microstructure through optimizing sintering conditions of the nanocomposite, such as sintering temperature,” they write.
In the second paper, published in September, the researchers used this new knowledge of SPS via glycine-nitrate processes to fabricate Y2O3–MgO ceramics doped with holmium (Ho3+: Y2O3–MgO).
Holmium-doped yttria is becoming an attractive option for producing eye-safe lasers operating at wavelengths of 2.0–2.2 μm due to the material’s inherently high efficiency and relatively simple thermal management. To date, researchers have achieved continuous-wave output power at 2,117 nm with a slope efficiency of 55.6% using 0.5 at.% Ho3+:Y2O3 ceramics. However, it is expected that Ho3+: Y2O3–MgO nanoceramics could result in further power scaling.
The researchers created 50:50 vol% Y2O3–MgO containing either 3, 6, or 12 аt.% Ho3+. They achieved the maximum value of Vickers hardness of 10.7 GPa for 12% Ho3+: Y2O3–MgO ceramics. In contrast, the highest in-line transmittance of 75% at 6-μm wavelength was obtained for ceramics doped with 3 at.% of Ho3+ ions, which is almost 5% higher transmittance compared to undoped Y2O3–MgO.
When these findings are taken into consideration with the luminescence intensity results, it “[proves] that Ho3+: Y2O3–MgO nanocomposite could be a promising material for high-power eye-safe lasers operating at wavelengths ~2 μm,” the authors write.
In a Far Eastern Federal University press release, Far Eastern senior research Denis Kosyanov says the light transmittance capacity should be increased from 75% to 80% for the nanocomposite ceramics to be used on the industrial scale. They plan to focus on this task at the next stage of their work.
The first paper, published in Ceramics International (April 2020), is “Influence of sintering temperature on structural and optical properties of Y2O3–MgO composite SPS ceramics” (DOI: 10.1016/j.ceramint.2019.11.137).
The second paper, published in Ceramics International (September 2020), is “A novel IR-transparent Ho3+: Y2O3–MgO nanocomposite ceramics for potential laser applications” (DOI: 10.1016/j.ceramint.2020.08.263).
Update 11/10/2020 – The incorrect name for Y2O3–MgO was initially stated. The correct name is yttrium magnesium oxide.