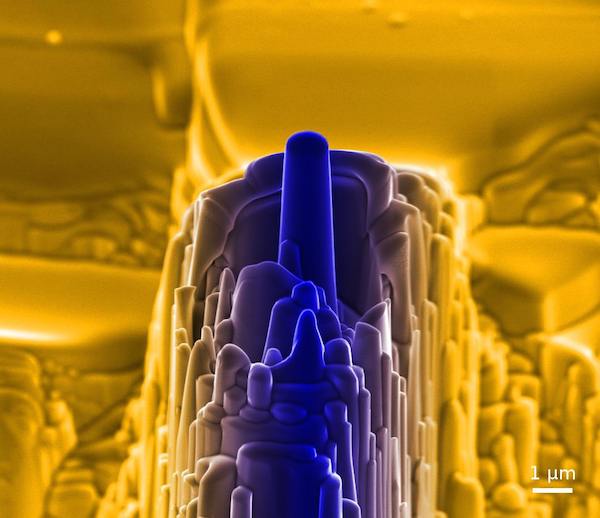
We go together, like…
…hardness and ductility?
When it comes to ceramics, the answer is not yet—but maybe soon.
Researchers at the University of California, Los Angeles have made new observations that could help design ultrahigh temperature ceramics that overcome the materials’ biggest limitation—brittleness. Their results are published as an invited feature in the Journal of the American Ceramic Society and don the cover of the journal’s August issue.
Ultrahigh temperature ceramics, as the name implies, have some of the highest melting points of known materials. And the materials also have a few added bonuses: high hardness, high strength, good wear resistance, high thermal conductivity, and good chemical and thermal stability.
Ductile versions of these super materials would be incredibly valuable in applications that demand strength and stability at high temperatures, such as aerospace applications.
The UCLA team tested transition-metal carbides zirconium carbide and tantalum carbide, observing how the materials atomically behaved under compression within a transmission electron microscope.
These transition metal carbides—along with other carbides and nitrides of group IV and V transition metals—have unique ionic, covalent, and metallic bonds that give the materials their useful properties. Transition metal carbides actually are more resistant to mechanical pressure and have a higher melting point than steel.
“Since the 1960s, if not earlier, researchers have studied transition-metal carbides,” says Suneel Kodambaka, UCLA associate professor of materials science and engineering and senior author of the new study, in an email. “Using rather simple, yet highly effective, measurement techniques, physical properties of these compounds have been determined. Researchers know that these materials are exceptionally hard. They also know that some of these compounds exhibit limited ductility. Despite this knowledge from ~50 years ago, we have not made much progress regarding the development of structurally tough transition-metal carbides.”
Kodambaka and his team—which included colleague Jenn-Ming Yang and graduate student Sara Kiani—sought to fill that knowledge gap. “The scientific driver for these tests was simple: determine the slip systems and gain fundamental understanding of the plastic deformation mechanisms operating at room temperature in these materials,” Kodambaka explains in the email.
By looking at just single crystals of zirconium carbide and tantalum carbide, the scientists noticed that the materials deformed under compression at room temperature. Importantly, the observations showed just how the crystals slip.
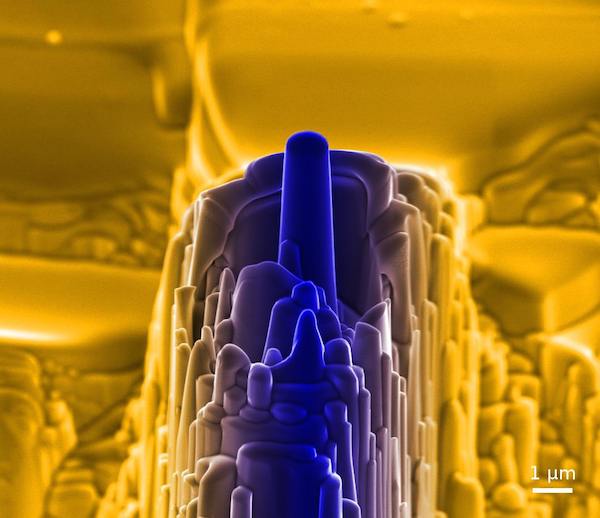
Electron micrograph of a micrometer-scale carbide pillar carved out of a single crystal using focused ion beams. Credit: Lawrence Berkeley National Lab
“Our experimental results reaffirmed what has been known in the community—that transition-metal carbides exhibit ductility,” Kodambaka says in the email. “Our new contributions to this existing field are as follows: 1) identification of easy slip systems operating at room-temperature; 2) these easy slip systems, contrary to popular belief, need not be the same ({110}<110>), as in rocksalt-structured ionic crystals, but depend on the transition-metal carbide; and 3) collection of crystal size-dependent yielding data, which provides new insights into possible mechanisms by which slip occurs in these crystals.”
So what do the observations mean?
“These results could help engineer the microstructures of ceramic components to tailor their mechanical properties,” Kodambaka says in a UCLA press release.
Via email, he elaborated a little further: “One can, in principle, use all of this new information to design polycrystalline transition-metal carbides with proper grain sizes and grain orientations that are most conducive for plastic deformation at room-temperature. It is also known in the literature that the mechanical properties of these carbides depend sensitively on their composition. Therefore, it is possible that, by choosing the appropriate alloying elements, ductility can be increased while retaining mechanical strength—i.e., make tough ceramics.”
But, Kodambaka, warns, that may not be as easy as he makes it seem.
“Both of these, however, are not trivial tasks and will require an iterative combination of multiscale modeling and experimental synthesis and testing studies to identify the optimal microstructures and compositions that will lead to high toughness. Probably an easier goal is the fabrication of thin (sub-micrometer) and flexible membranes made of these materials for miniature structural components for MEMS and NEMS devices.”
However, the possibilities stretch much further. Ductile-yet-hard ceramics would be superior materials for aerospace applications of all sorts, radiation-resistant foils for solar sails, and more.
To Kodambaka, the research has been an exciting example of scientific discovery.
“For me, the exciting part of this research was the fact that we were able to find something new and surprising using conventional uniaxial compression tests on single crystals of ZrC and TaC. There are several other crystal orientations and other transition metal carbides on which such studies could be conducted and new phenomena discovered. Couple this with the fact that these carbides can exist as sub-stoichiometric compounds (i.e., C-vacancies) with stoichiometry-dependent properties and that one could synthesize myriad alloys of these carbides, possibilities of finding new information are endless.”
The paper is “Nanomechanics of refractory transition-metal carbides: A path to discovering plasticity in hard ceramics” (DOI: 10.1111/jace.13686).