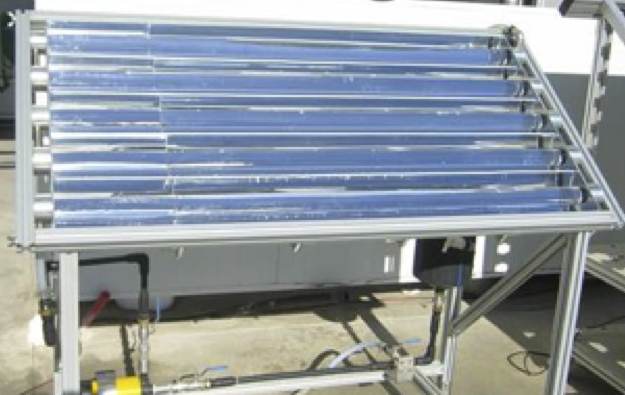
Pilot-scale photo reactor for water disinfection at Plataforma Solar de Almería, Spain. (Credit: Pillai; RSC)
I live in the Great Lakes region of the United States. Like the millions who live in this region of the Midwest, I do not worry about access to clean, potable water in the near- or far-term.
However, millions of people throughout the world do worry about access to water. Nearly 800 million people throughout the world live without access to clean water, according to the nonprofit, Water.org. And, nearly 2.6 billion people live in areas without improved sanitation, further exacerbating the crisis. (Water.org was founded by Gary White, a Missouri S&T alum, and Matt Damon—yes, that Matt Damon.)
Access is only the first concern. Water in many regions is contaminated by organic pollutants or contains harmful pathogens. The water crisis disproportionally affects women (who spend an estimated 200 million hours per day getting water) and children, who are more susceptible to water-borne diseases.
In 2010, the United Nations declared access to clean water and sanitation a fundamental human right and set a goal (pdf) of reducing by half the population around the globe without access to potable water and basic sanitation by next year, 2015.
Sadly, poor water quality and poverty tend to go hand-in-hand, so any remedy needs to be inexpensive, independent of infrastructure (such as power), and easy to implement. Portability is an advantage, too. The UN goals are to ensure that 50–100 liters of water per person per day are available within 1,000 m of their home. It should take less than 30 minutes to collect water, and the water should not cost more than three percent of household income. (Ask yourself, would you walk a kilometer for water to brush your teeth? Would you pay up to three percent of your income for water?)
Sunshine tends to be an abundant resource in regions suffering most from poor water quality. Photocatalytic materials, such as semiconducting TiO2 and ZnO, activated by sunlight can help meet UN clean water goals. A recent paper in the journal Catalysis Science & Technology (subscription required) reviews the current status of solar disinfection (SODIS) technology. (Ironically, most of the paper’s authors hail from Ireland, known more for its citizens’ sunny dispositions, despite the lack of sunny climate.)
The review focuses on advances for using titania photocatalyst and design of disinfectant reactors, either as bottles or as plant facilities. Titania’s (anatase form) photocatalytic property is activated by high wavelength ultraviolet light. In order to maximize efficiency, its photocatalytic property must activate in the visible region of the solar spectrum, too, which means reducing the band gap of titania. One approach is to dope with nitrogen or copper, according to the paper.
Corresponding author Suresh Pillai, senior lecturer in environmental sciences at Institute of Technology Sligo (Sligo, Ireland) says mass transfer also limits efficiency. He says in an email that “mass transfer [of bacteria to the active surface] has long been identified as the major limitation in applying the intrinsic advantage of photocatalytic water decontamination.” According to the paper, nanotechnology may help address this constraint, “Nano-assembled materials (such as nanoparticles, nanotubes, nanofibers, nanocages, nanorods, etc.) have been shown to enhance the photoactivity of TiO2, with the key contributing factor being the specific surface area of the structure.”
Pillai says smaller coated bottles perform better than larger ones – again because of the limitations of surface area. The paper points out another challenge. The photocatalytic activity occurs when the water, light, and titania interact inside the bottle. Thus, bottle interiors must be coated such that they do not block light from transmitting into the bottle.
The review paper looks at design of reactor systems and their efficiency and efficacy. Similar to solar collectors, they take advantage of compound parabolic mirror troughs to harvest and focus solar irradiation. According to the paper, these systems have low environmental impact, including minimal power requirements, and are easy to construct and maintain. The paper cites one study that estimates a 25-liter batch reactor “could provide solar disinfected water at a total treatment cost of $0.20 per 100 l, taking into account that the estimated photoreactor built cost is $200 with 10 years of operational life.”
That price point approaches the UN’s three percent of income goal in some regions, but is still far too high for many water-crisis countries. Twenty cents per 100 liters meets the target for annual incomes of about $2,550 per year. According to International Monetary Fund estimates for 2013 GDP, India, Ghana, and Laos ($4,077–$3,068) meet that price criterion. Countries such as Nigeria, Sudan, Cambodia, and Chad ($2,831–$2,539) flirt with the borderline. Too many countries do not come close: Sierra Leon, Rwanda, Uganda, Ethiopia, South Sudan, Haiti, Zimbabwe, Burundi, ($1,542–$642) and more. For perspective, United States 2013 GDP is estimated at $53,101.
Pillai says he knows of at least one commercial-scale reactor, located in Spain (pictured above). Despite numerous lab studies, he says commercialization of SODIS has been “remarkably low.” He notes, “This is surprising considering that in 2009, two million users were practicing solar disinfection in 33 countries.”
Besides providing the usual overview of state-of-the-art that one expects from a review paper, this paper provides a roadmap for addressing remaining technological challenges, in particular, mass transfer, bottle reactor design, and standardized tests for evaluating water quality (with respect to organic compound breakdown as well as microorganism annihilation).
It is raining today as I write this, and my neighborhood Great Lake is being replenished. I am grateful.
The paper is “Solar photocatalysis for water disinfection: materials and reactor design,” by Keane, et al. (DOEI: 10.1039/c4cy00006d)