[Image above] A large-area elemental map (calcium: red, silicon: blue, aluminum: green) of a 2 cm fragment of ancient Roman concrete (right) collected from the archaeological site of Privernum, Italy (left). A calcium-rich lime clast (in red), which is responsible for the unique self-healing properties in this ancient material, is clearly visible in the lower region of the image. Credit: Seymour et al., Massachusetts Institute of Technology
For all our current technological innovations, sometimes scientists of the past achieved feats that matched or surpassed our modern efforts. While some of these achievements have yet to be replicated—such as the Blaschka invertebrate glass models—modern characterization tools have revealed the secrets of other historical marvels, such as Roman concretes.
Compared to contemporary concretes, which tend to deteriorate within decades, Roman concretes have remained coherent and well-consolidated for more than 2,000 years, even in aggressive maritime environments.
The key to Roman concrete durability is its use of volcanic ash and other natural pozzolans as cementitious materials rather than today’s Portland cement. When mixed with lime and water, these materials react and form a 3D clast-supported framework that resists displacement and fracture better than Portland cement-based structures.
Numerous studies on Roman concretes, many of which were led by ACerS Fellow Marie Jackson, have revealed insights into the various chemical compositions and formation mechanisms. Yet even with decades of research, scientists are still making new discoveries concerning the properties of these concretes. For example, the role that aggregate-scale lime clasts play in the durability of Roman concretes.
These distinctive bright white clasts, also called remnant lime or lime lumps, are a ubiquitous and conspicuous feature of both land-based and maritime Roman concretes. Traditionally, researchers have considered these clasts “relicts” of the concrete fabrication process, which results from incomplete or over-burning during the calcining of lime, carbonation before concrete preparation, incomplete dissolution during setting, or insufficient mixing of the mortar.
This “relict” characterization of the lime clasts bothered ACerS member Admir Masic, professor of civil and environmental engineering at Massachusetts Institute of Technology.
“If the Romans put so much effort into making an outstanding construction material, following all the detailed recipes that had been optimized over the course of many centuries, why would they put so little effort into ensuring the production of a well-mixed final product?” he says in an MIT press release.
Spurred on by this question, Masic and colleagues at MIT and Harvard University, along with researchers at laboratories in Italy and Switzerland, took a closer look at the clasts. According to their analysis, the inclusion of these clasts may not be so accidental after all.
Their study focused on 2,000-year-old Roman concrete samples obtained from the archaeological site of Privernum, Italy. The samples were sourced from the masonry mortar of the city wall.
Spectroscopic examination of the samples provided clues that the concrete was formed at extreme temperatures. This finding is significant because it suggests the Romans employed a hot mixing technique—introducing quicklime directly into the mixture—rather than slaking, i.e., first hydrating the quicklime before introducing it. Such an approach would “create an environment where high surface area aggregate-scale lime clasts are retained within the mortar matrix,” the researchers write.
But why would the Romans want to use an approach that purposefully retains lime clasts within the mortar matrix? The researchers suggest these clasts may provide the concrete with a self-healing ability.
“The recent discovery of calcite-filled cracks in Roman concrete suggested a potential long-term healing process that requires a Ca-rich source,” the researchers write. “Considering the ubiquity of relict lime clasts in Roman concrete and their high surface area due to their particulate microstructure, these inclusions might provide the requisite Ca reservoirs for these processes.”
To test this hypothesis, the researchers created cementitious formulations based on the Roman concretes. After curing the modern samples, they fractured the samples and then flowed water over them for 30 days.
Compared to the clast-free control samples, water stopped flowing through the clast-containing samples within a month. Examination of the cracked surface revealed it had filled completely with a newly precipitated mineral phase.
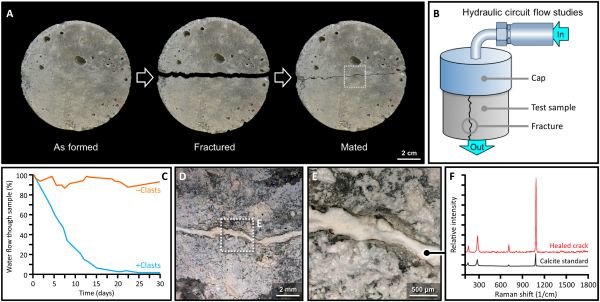
After casting, the Roman-inspired hot-mixed concrete samples were mechanically fractured and then remated (with a gap of 0.5 ± 0.1 mm) and preconditioned for the crack-healing studies (A). Using an integrated flow circuit (B), water flow through the sample over the course of 30 days was documented with a flow meter. Compared to the lime clast-free control (orange line), after 30 days, water flow through the lime clast-containing sample (blue line) ceased (C), and examination of the cracked surface revealed that it had been filled completely with a newly precipitated mineral phase (D and E), which was identified as calcite from Raman spectroscopy measurements (F). Credit: Seymour et al., Science Advances (CC BY-NC 4.0)
As a result of these successful tests, the MIT press release reports that the researchers are working to commercialize this Roman-inspired concrete.
“It’s exciting to think about how these more durable concrete formulations could expand not only the service life of these materials, but also how it could improve the durability of 3D-printed concrete formulations,” Masic says in the press release.
The open-access paper, published in Science Advances, is “Hot mixing: Mechanistic insights into the durability of ancient Roman concrete” (DOI: 10.1126/sciadv.add1602).
Author
Lisa McDonald
CTT Categories
- Art & Archaeology