[Image above] Researchers at Drexel University have observed a new type of structural deformation mechanism in bulk materials, called ripplocation, that occurs when atomic layers inside the material ripple and buckle during compression. Credit: Drexel University
New research is maximizing on the potential of MAX phases, a group of layered ternary carbide and nitride materials with a unique combination of ceramic and metal properties.
[For more information on MAX phases, see this 2013 ACerS Bulletin article by Miladin Radovic and Michel Barsoum.]
Last week I reported on 2-D MAX phase materials, called MXene materials, that can provide superior electromagnetic shielding with less bulk than conventional metal strategies for protecting electronic devices.
And now researchers at Drexel University report on their studies of MAX phase ceramics that describe a completely new observation of how materials deform—a finding with broad implications for various other kinds of layered materials.
Understanding how materials deform is important to understanding their behavior. Sure, we know that dislocations or defects in a material are integral to how that material deforms. But we still don’t understand a lot about how layered materials respond to deformation.
“Dislocation theory—in which the operative deformation micromechanism is a defect known as a dislocation—is very well established and has been spectacularly successful in our understanding the deformation of metals,” Garritt Tucker, an assistant professor of materials science and engineering at Drexel University, says in a Drexel news release. “But it never really accurately accounted for the rippling and kink band formation observed in most layered solids.”
Dislocation theory predicts that, when deformed edge-on, the planes of a layered solid material either will become indented or, if elastic, will bounce back to the original form.
But layered materials don’t quite do either—although they can bounce back, they do so while forming permanent kink bands. Michel Barsoum, Drexel distinguished professor of materials science and engineering and head of the Drexel MAX/MXene Research Group, described this effect about a decade ago, then calling it “kinking nonlinear elasticity” (for more information, see the 2013 Bulletin article, which Barsoum co-authored, mentioned above).
Just last year, a separate MIT group published a paper describing a new phenomenon of deformation in the surface layers of 2-D materials. Dubbed “ripplocations”—a word mashup between surface ripples and crystallographic dislocations—this option describes how deformed layered materials form internal atomic scale ripples that dissipate energy.
“The MIT work showed that while the end result of the motion of dislocations and ripplocations is the same—one atomic layer moves relative to another—their physics were distinctly different and were thus totally and fundamentally different entities,” Barsoum explains in the Drexel release.
The idea of ripplocations fit well into Barsoum’s working theory of how kinking nonlinear elastic behavior works within dislocation theory—but could the same 2-D layer phenomenon observed at MIT hold true for layers in bulk materials?
To find out, the Drexel scientists first performed simulations on the familiar layered material graphite, and then extended their studies to indentation experiments with samples of MAX phase ceramic Ti3SiC2.
“We ran atomistic simulations on a bulk sample of graphite, because it is a layered material that has been studied quite a bit and it is used in a number of applications where it is loaded,” Jacob Gruber, first author of the new paper and Drexel doctoral candidate, says in the release. “By constraining the edges of the sample while compressing the material, we observed the nucleation and motion of a multitude of ripplocations that self-assemble into kink boundaries. The observation is significant because these are the same sort of kink bands that are ubiquitous in geologic formations and layered solids that have been deformed.”
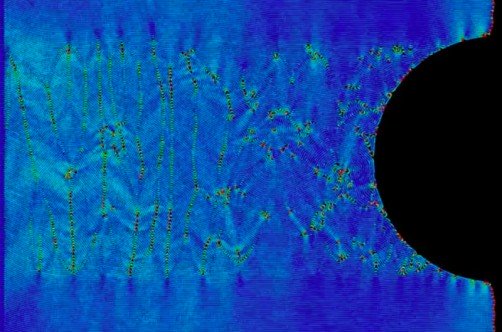
When a layered material like graphite receives an edge-on force, it can elastically recover its shape because the force is dissipated through ripplocation of its internal atomic layers. Credit: Drexel University
After the simulations indicated how the layered materials would behave under confined compression—a response that resembled a buckling phenomenon, the authors write in the paper’s abstract—the team then used a spherical indenter to see if the predictions held true in experimental samples of MAX phase ceramic Ti3SiC2.
“When we obtained high resolution transmission electron microscope images of the defects that formed as a result of the deformation, we were not only able to show that they were not dislocations, but as importantly, they were also consistent with what ripplocations would look like,” Mitra Taheri, Hoeganaes associate professor of materials science and engineering at Drexel, says in the release. “We now have evidence for a new defect in solids; in other words, we have doubled the deformation micromechanisms known.”
And those deformation micromechanisms extend to a lot of different layered materials—meaning important and broad applicability of the experimental results.
“There are many layered solids, in both nature and the built environment, that are technologically important, so it’s essential to understand their behavior,” Barsoum adds in the release. “This new finding will require us to reexamine past findings and reinterpret results that to date were incorrectly explained using dislocation theory.”
Check out this series of Youtube videos to see the researchers’ simulations of the ripplocation phenomenon.
The open-access paper, published in Scientific Reports, is “Evidence for bulk ripplocations in layered solids” (DOI: 10.1038/srep33451).
Author
April Gocha
CTT Categories
- Basic Science
- Material Innovations
- Modeling & Simulation