Making the lithium-ion batteries that power everything from hybrid and all-electric vehicles to cell phones and other mobile electronic devices safer, longer lasting, and less expensive is a key goal for researchers in the field.
Conventional Li-ion batteries use a liquid conducting medium to allow current to flow between the battery’s anode and cathode. Scientists at Michigan State University (E. Lansing) are among several groups working to change that by developing solid conductors with current-conducting capability the same as or better than that of liquid conductors.
“We’re working to create the next generation of batteries for electric vehicles,” says researcher Jeff Sakamoto. “If you want to eliminate ‘range anxiety’ and sticker shock, you must have a battery that stores a lot more energy—four or five times more—and cost about a fourth or fifth of what lithium-ion batteries cost today.”
According to Sakamoto, assistant professor of chemical engineering and materials science, a class of ceramics called superionic conductors is among the most promising candidate solid conductor materials. “The goal is to move away from liquid cells and toward solid-state batteries that are safer, cheaper to manufacture, less sensitive to degradation at higher temperatures, and more durable,” Sakamoto says in this news release.
Ions move throughs superionic conductors just as efficiently as through liquid electrolyte—at ~1 mS/cm at room temperature, according to Sakamoto. “In a typical superionic conductor, a stable ceramic oxide or sulfide primary lattice provides a ‘skeleton framework’ that allows a highly mobile sublattice of cations, in this case lithium ions, to move freely,” he explains in an email.
The MSU team is studying ceramic oxide superionic conductors with the garnet mineral structure and nominal composition of Li7La3Zr2O12. Sakamoto says he has been working with this material, along with Jeff Wolfenstine of the Army Research Lab in Adelphi, Md., for about four years.
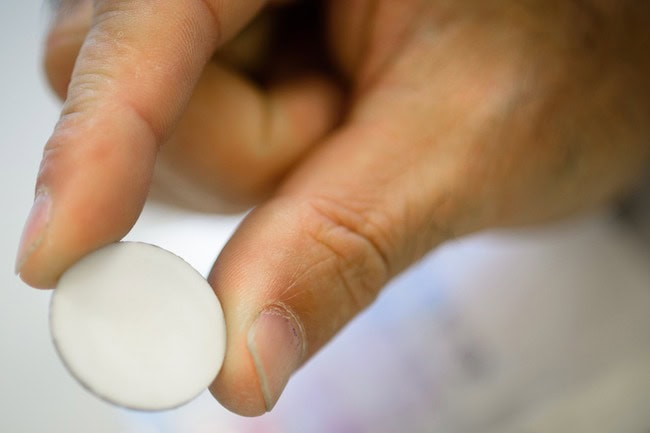
Rapid induction hot pressing technique developed at MSU results in test membranes of ~98% theoretical density to enable fundamental studies. Credit: G.L. Kohuth/MSU.
“I am excited about this material because it has a unique combination of superionic conductivity and stability against lithium,” Sakamoto says. “The latter opens the electrochemical stability window to enable metallic lithium or other advanced anode materials.”
The MSU group has synthesized the material in powder form through solid state reactions and a sol–gel alkoxide method, and now is using a “simple, cheap, scaleable water-based technique,” Sakamoto says. (The large photo above shows Travis Thompson, a doctoral student in materials science and engineering, preparing to synthesize the material. Credit: G.L. Kohuth/MSU.)
“Once we obtain the powders, we fabricate and densify membranes through a rapid induction hot pressing technique that we developed,” he continues. “Although it may not be scaleable, RIHP does produce membranes in close to the ideal form (~98% theoretical density) to enable fundamental studies.”
Sakamoto says the garnet material was first reported by a German research group several years ago, and that researchers at Toyota, BASF, Bosch, NGK, Samsung, and other companies are also working with the material.
“My group has investigated new areas such as synthesis, stability, and device integration,” he says. “I am optimistic that this material has the potential to enable numerous new energy storage technologies such as solid-state, redox-flow, lithium-sulfur, and lithium-oxygen energy storage technology.”
Author
Jim Destfani
CTT Categories
- Electronics
- Energy