[Image above] Atomic structure of a layered oxide material designed by James Rondinelli’s research group. Credit: Northwestern McCormick School of Engineering
As a writer, editor, and lover of words, I’m rather fond of grammar and word usage.
I’m a slasher of unnecessary articles. (I’m looking at you, the). I’m zealous about the difference between that and which. I’m the Oxford comma’s biggest fan.
And I’m also rather easily amused—I like it when small changes drastically alter the meaning of words and phrases.
Case in point: the band gap and The Gap Band.
The same three words, rearranged ever so slightly, cull drastically different results from omniscient Google.
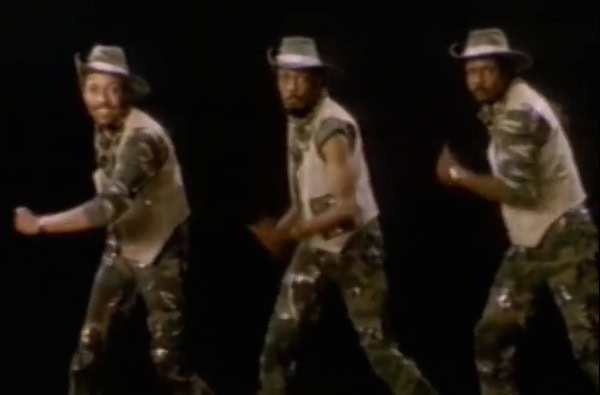
The Gap Band. Credit: TheGapBandVEVO; YouTube
Although you could spend several minutes of your life watching 1980s music videos from The Gap Band, you probably have work to do. So let’s talk about the band gap instead.
ACerS member James Rondinelli, a materials science and engineering professor at Northwestern University’s McCormick School of Engineering, and his research group are studying how to adjust the electronic band gap in complex oxides by simply adjusting a material’s properties, rather than its overall composition.
Tuning band gaps has been covered on CTT before. That’s because it’s an important, although challenging, feat—a material’s band gap dictates its properties and so its potential applications, too.
A band gap is kind of like a no-fly zone for electrons—it’s the amount of energy needed for an electron in a semiconductor to move from a bound state to a free state—from a valence band to a conduction band. In the conduction band, electrons can conduct energy.
Band gaps are especially important because they dictate how a material harvests and converts light, and thus a material’s solar energy capabilities.
“There really aren’t any perfect materials to collect the sun’s light,” Rondinelli says in a Northwestern press release. “So, as materials scientists, we’re trying to engineer one from the bottom up. We try to understand the structure of a material, the manner in which the atoms are arranged, and how that ‘genome’ supports a material’s properties and functionality.”
Rondinelli’s team uses quantum mechanics calculations to figure out how to change a material’s band gap by examining how the layers within an oxide interact with one another.
“Today it’s possible to create digital materials with atomic level precision,” Rondinelli says in the release. “The space for exploration, however, is enormous. If we understand how the material behavior emerges from building blocks, then we make that challenge surmountable and meet one of the greatest challenges today—functionality by design.”
That goal aligns closely with those of the Materials Genome Initiative (more coverage here), the White House initiative “to discover, manufacture, and deploy advanced materials twice as fast, at a fraction of the cost.”
Rondinelli’s team’s simulations controlled interactions between layers of complex oxides, specifically between neutral and charged layers. Tuning the arrangement of cations tuned the material’s overall band gap without altering the material’s composition.
The team’s computations showed that they could adjust the oxides’ band gap by more than two electronvolts. Conventional methods to tune band gaps—which also require adjusting a material’s composition—can only change the band gap by about one electronvolt, according to the press release. The next challenge is to test the computations with experiments.
“You could actually cleave the crystal and, at the nanometer scale, see well-defined layers that comprise the structure,” Rondinelli says in the release. “The way in which you order the cations on these layers in the structure at the atomic level is what gives you a new control parameter that doesn’t exist normally in traditional semiconductor materials.”
Adjusting the band gap means new properties for the material, opening the possibility for engineering future materials to be more precisely fine-tuned to specific applications.
“The finding could potentially lead to better electro-optical devices, such as lasers, and new energy-generation and conversion materials, including more absorbent solar cells and the improved conversion of sunlight into chemical fuels through photoelectrocatalysis,” according to the release.
The paper, published in Nature Communications, is “Massive band gap variation in layered oxides through cation ordering,” (DOI: 10.1038/ncomms7191).
Author
April Gocha
CTT Categories
- Electronics
- Energy
- Material Innovations
- Modeling & Simulation