[Image above] Concrete walls of Trajan’s Markets in Rome, which have stood tall for nearly 2,000 years. Credit: Marie Jackson
Last spring, I lamented about the crumbly-cookie status of concrete steps descending from my home’s back door (we’ve written a lot more about concrete since then, too).
Though my home is officially listed on the county auditor’s website as being built in the year “OLD,” (due to a lack of records pre-1915), the concrete steps were a much more recent addition—yet they still have trouble holding shape against Ohio’s seasonal temperature mood-swings.
And yet ancient concrete structures like the Pantheon and Coliseum in Rome, Italy, still stand strong and tall despite a couple thousand years of wear. What’s the difference between their concrete and mine?
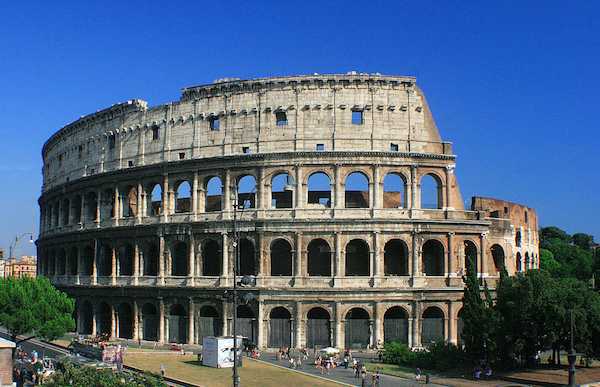
The Coliseum in Rome—still standing. Credit: Valdiney Pimenta; Flickr CC BY 2.0
For starters, Roman concrete was not only strong and durable, but also much more environmentally friendly than the ubiquitous Portland cement used today. That’s because Portland cement production requires heating a limestone–clay mixture to 1,450°C, releasing a lot of carbon in the process—enough to account for 7% of global carbon emissions in total.
Roman construction instead used large chunks of rock (45–55% by volume) bound together by a mortar composed of 85% by volume volcanic ash mixed with water and lime, a formulation that requires much lower production temperatures and thus lower carbon emissions, too.
As previously reported on CTT, a study led by University of California, Berkeley, volcanologist and scientist Marie Jackson already found that Roman concrete’s binder contained aluminum and has less silicon in comparison to the calcium, silicate, and hydrate mixtures of modern concrete binders. That aluminum content, in the form of aluminum tobermorite, a rare hydrothermal mineral, bestowed the old concrete with higher stiffness, the researchers thought.
A new study led by Jackson confirms that unique aluminum-containing crystals that form in Roman concrete are behind the material’s robust strength and durability. The results, published in the Proceedings of the National Academy of Sciences (PNAS), are particularly interesting to efforts to make modern concrete more durable and more sustainable.
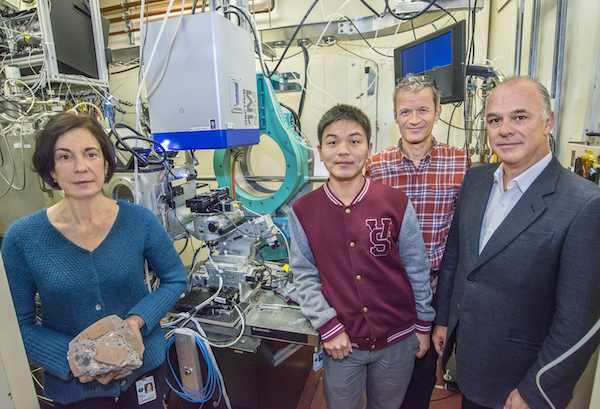
(From left) Marie Jackson, Qinfei Li, Martin Kunz and Paulo Monteiro at ALS Beamline 12.3.2. Credit: Roy Kaltschmidt; Berkeley Lab
“If we can find ways to incorporate a substantial volumetric component of volcanic rock in the production of specialty concretes, we could greatly reduce the carbon emissions associated with their production and also improve their durability and mechanical resistance over time,” Jackson says in a Lawrence Berkeley National Lab press release.
To get a better look into concrete’s structure, the team examined its mortar using synchrontron X-rays. We recently told you a story (on CTT back in August, and more in-depth in our print publication, the Bulletin, in the January/February issue available now) about how synchrotron X-rays have helped researchers probe the secrets of thermal barrier coatings for jet engines (ACerS members can download that article—for FREE!—here). Now synchrotron X-rays are helping to shed light on the ancient Roman concrete, too.
The scientists convened at Berkeley Lab’s synchrotron, the Advanced Light Source, at beamline 12.3.2 to use X-rays to look within 0.3 mm-thick slices of Roman mortar. “We obtained X-ray diffractograms for many different points within a given cementitious microstructure,” Jackson says in the release. “This enabled us to detect changes in mineral assemblages that gave precise indications of chemical processes active over very small areas.”
The team put together analyses of both a reproduction of ancient concrete and a sample of the real deal, in an effort to understand what was happening within the concrete. “Through observing the mineralogical changes that took place in the curing of the mortar over a period of 180 days and comparing the results to 1,900 year old samples of the original, the team discovered that a crystalline binding hydrate prevents microcracks from propagating,” according to the release.
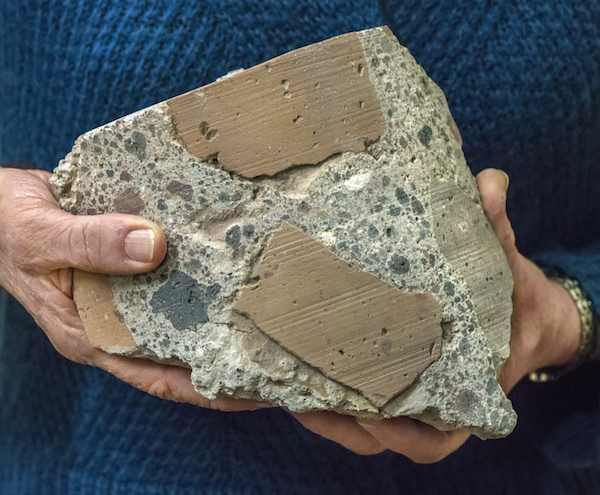
Ancient Roman concrete consists of coarse chunks of volcanic tuff and brick bound together by a volcanic ash-lime mortar that resists microcracking, a key to its longevity and endurance. Credit: Roy Kaltschmidt; Berkeley Lab
During curing, Roman concrete’s calcium-aluminum-silicate-hydrate binder got stronger and tougher thanks to the growth of platy strätlingite crystals in between the volcanic material and the mortar.
“The mortar resists microcracking through in situ crystallization of platy strätlingite, a durable calcium-alumino-silicate mineral that reinforces interfacial zones and the cementitious matrix,” Jackson says in the release. “The dense intergrowths of the platy crystals obstruct crack propagation and preserve cohesion at the micron scale, which in turn enables the concrete to maintain its chemical resilience and structural integrity in a seismically active environment at the millennial scale.
These same structures aren’t present in Portland cement, which instead has high porosity at those interfaces that allows cracks to form and propagate.
The paper is “Mechanical resilience and cementitious processes in Imperial Roman architectural mortar” (DOI: 10.1073/pnas.1417456111).
Author
April Gocha
CTT Categories
- Art & Archaeology
- Basic Science
- Cement
- Construction
- Energy
- Environment
- Material Innovations