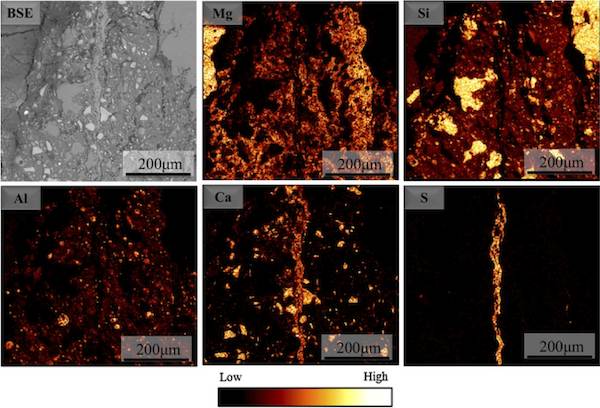
Sheffield University professor John Provis on the durability of “geopolymer” cement, a possible substitution for clinker-based cements. Credit: Wiley; YouTube.
Concrete—aggregate bound together with cement—has been the “go to” building material since ancient times, and evidence suggests that the Romans developed standardized formulations for making cement and mixing concrete. The Romans knew what they were doing—the Pantheon, which was built during the reign of Roman emperor Augustus (27 BC–14 AD), is the largest unreinforced concrete dome in the world despite being more than 2,000 years old.
Concrete is as important as ever and is the most widely used material in the world. Concrete use is even used as an indicator of economic growth, especially in developing regions.
According to an International Cement Review presentation last fall (pdf), the amount used in the decade spanning 2002–2012 doubled from 1.8 billion tons to 3.7 billion tons. The industry contributes about 5% of anthropogenic CO2 worldwide, and thus gets a lot of attention. However, the industry has been proactively addressing the issue for quite some time. EnvironmentLeader.com reported last summer that statistics from the Cement Sustainability Initiative indicate CO2 emissions since 1990 have dropped from 756 kg/ton to 629 kg/ton—a 17 percent reduction.
Any chance of less concrete being used? Nope.
Karen Scrivener explains in a Chemistry World article (pdf), “The reason there’s so much concrete is because it is in fact a very low impact material.” She says substituting other materials for concrete would only lead to much higher CO2 emissions. “The reason concrete has a big carbon footprint as a whole is that there are just such huge quantities used,” she says. Scrivener leads the construction lab at the Swiss Federal Institute of Technology, Lausanne.
A report from the National Ready Made Concrete Association (pdf) supports Scrivener’s claim, citing data from a study published in the American Concrete Institute Materials Journal. At the time of the study in 1997, production of portland cement consumed one-sixth as much energy as steel. We know the efficiency of both industries has improved significantly in the intervening 17 years, however, the embodied energy of steel still far outpaces concrete.
That does not remove the onus from the concrete industry to lead the effort to reduce its carbon footprint. And, research into alternative, low-carbon cements and alternatives to portland cement comprise a lively, active field of inquiry. Developing low-clinker content cements is a key focus, according to a report from the European Cement Association (pdf).
A group out of Sheffield University in the UK reported recent findings on their work developing clinker-free cements for concrete in the April issue of the Journal of the American Ceramic Society (subscription required, but view abstract here). They call these compounds “alkali-activated materials (AAM),” however, these compounds have been known by many other names, perhaps most famously as “geopolymers.” In the video above, coauthor, SU professor, and ACerS member John Provis summarizes the work and its importance. The lead author is Susan Bernal, a research fellow at SU.
Any substitute for portland cement must be producible throughout the world from a wide range of starting materials. Also, the material properties must be comparable in the fresh state—so that the concrete can be worked with existing tools and skill sets—and in the hardened state.
AAMs result from a reaction between an alkali source and a pozzolanic aluminosilicate precursor, such as blast-furnace slag, fly ash, or calcined kaolinite minerals. The precorsors contain reactive aluminum and silicon, which dissolve under the right alkaline conditions to form a cement-like binder and then rearrange through solution to form a strong dense binding gel.
The authors are careful to note that an all-purpose substitute for portland cement is unlikely. Thus, the chemical and physical properties, that is, the durability of alternative materials, will dictate applications.
The authors report on the gel chemistry and how it varies with calcium content. Also, these materials are “highly spatially heterogeneous,” reflecting inhomogeneity of precursor material particle size, chemistry, mineralogy, and reactivity. That means microstructure, including porosity, dictates properties and must be understood.
The durability discussion in the paper addresses curing, weathering and chemical attack (carbonation), sulfate exposure, and chloride exposure (especially important for steel reinforced concrete).
The authors conclude that “durability of AAMs is strongly dependent on the nano- and microstructure of the reaction products forming in these systems, as a function of the type of precursor, the nature and concentration of the activator, and also the maturity of the material. High-Ca systems have a structure mainly dominated by a C–A–S–H gel, which is less porous than the ‘geopolymer’ gel forming in low-Ca systems. This is one of the main factors controlling the transport properties of AAMs; however, the differences in chemistry of both pore solution and reaction products in AAMs produced with different precursors modify the chemical mechanisms that can lead to the decay of these binders when exposed to aggressive environments.”
They also say, however, that the mechanisms of structural changes need to be better understood before AAMs are used, especially how they interact with different environments. Developing models and tests to predict service life in specific environments will be important for developing AAM cements and making progress toward formulating clinker-free concrete.
The paper is “Durability of alkali-activated materials: Progress and perspectives,” by Susan A. Bernal and John L. Provis. (DOI: 10.1111/jace.12831).
ACerS members have free access to all of ACerS journals. Learn more about becoming a member.
Featured image above: BSE image and elemental maps of alkali-activated 75 wt% slag/25 wt% fly ash concrete with 28 d curing after 6 months of MgSO4exposure (Courtesy of I. Ismail; JACerS, Wiley.)