[Image above] Droplet of molten uranium dioxide suspended in a levitation stage for X-ray diffraction studies. The three millimeter-wide droplet is melted with lasers. Credit: Argonne National Laboratory
March 11, 2011 seems like a long time ago, but odds are you remember watching with horror as a historic tsunami struck eastern Japan that day. Tsunmai waves that sloshed over the nuclear power plant at Fukushima-Dai-ichi reached heights twice the “worst case” engineers had designed for and overwhelmed multiple backup systems for keeping the reactor core cool.
Absent cooling systems, thermodynamics dictated a sequence of materials reactions leading to inevitable disaster. Within a day, the zircaloy cladding in reactor 1 failed and reacted with pumped-in seawater to yield hydrogen, which exploded dramatically. The core, too, melted and fell to the bottom of the reactor. Later, cores of reactors 2 and 3 would also fall to the bottom of their reactors.
In the aftermath, some countries decided to eschew nuclear power. However, nuclear energy still provides about 10 percent of the world’s electricity and avoids pumping an estimated 64 x 1012 kg of CO2 emissions into the atmosphere.
Like it or not, nuclear power holds a permanent place in the global energy portfolio. However, events such as the tsunami and Chernobyl remind us of the importance of safety. Researchers are addressing safety—always paramount in the nuclear industry—on several fronts.
For example, new reactors designed with “passive” cooling systems are one solution. However, most already-deployed systems do not have passive cooling systems. If the worst happens, the guys in the control room need to know what is happening with the materials in a hot, dynamic environment. And the one they care most about is the radioactive fuel—uranium dioxide.
A new article in Science (subscription required) characterizes for the first time the structure of UO2 in its molten and hot, solid state (slightly below melting).
Coauthor and ACerS member Richard Weber, says, “One takeaway from the new work is that while models of liquid structure are valuable to help design safe reactors, benchmark measurements on real materials are essential to validate the models. By measuring actual fuel material, this work provided a key missing part of the story.”
Uranium dioxide is not easy to work with. Highly refractory, it melts (pdf) at about 2,800˚C. At 2,400˚C it undergoes a “superionic lambda transition” that involves a rapid disordering of the oxygen sublattice. As temperatures approach the lambda transition, heat capacity and thermal conductivity rise steeply. Uranium and zirconium eutectics occur in the 2,500–2,700˚C range, adding to the high-temperature messiness.
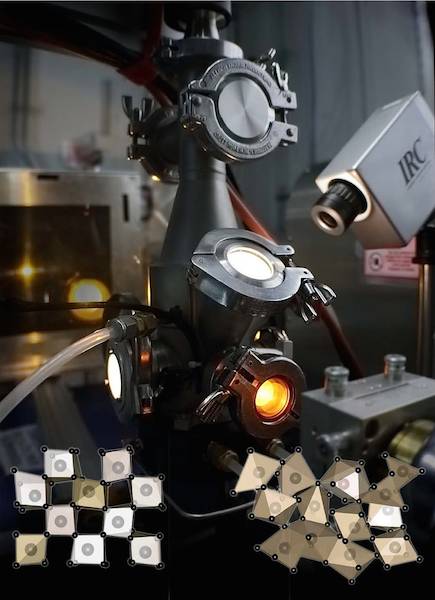
An Argonne-led research team found that when uranium dioxide melts, the number of oxygen atoms around uranium changes from eight-fold to a mixture of six- and sevenfold, which alters how it interacts with other materials. Credit: Argonne National Laboratory; Flickr CC BY-NC-SA 2.0
Modeling of molten structure has produced inconsistent results, mostly because models rely on data from solid-state properties. Accurate knowledge of the melt structure is critical, writes University of California, Davis, professor Alexandra Navrotsky in a “Perspectives” piece in the same Science issue, because it determines physical properties such as viscosity, rheology, thermal expansion, thermal conductivity, etc. She notes that molten uranium oxide can dissolve species from other reactor components, such as zirconium from zircalloy cladding, iron from steel containments, and calcium, aluminum, and silicon from concrete.
Models did not describe hot solid structure, either. For example, the uranium–oxygen bond length shrinks near the melting point because of increasing disorder of the oxygen sublattice. The uranium–uranium bond expands enough to cause an overall volume expansion as the melting point is approached. Below the lambda transition, oxygen and uranium diffusion is on the same order of magnitude. But, above it, oxygen diffusion is 10,000 times faster. This leads to a disordered and dynamic crystal structure, loaded with oxygen and interstitial vacancies.
Weber was part of a team based at Argonne National Laboratory that used the lab’s Advanced Photon Source combined with a levitation stage (which we wrote about in CTT a few months back) to conduct X-ray diffraction measurements on molten UO2. (Weber’s company, Materials Development Inc., designed the levitation stage, which helped to greatly expand the temperature range over which molten oxides can be studied.) Lasers melted UO2 spheres, and the levitation stage eliminated contact contamination.
Experiments showed that the UO2 melt contains U-O polyhedra with several coordination structures, comprising a random mix of primarily UO7, UO6, UO8, and UO5. The polyhedral do not cluster, and the mix of species is dynamic and temperature-dependent. Navrotsky says, “It is reasonable to speculate that the liquid could readily incorporate other components.”
With accurate data to validate and modify models, nuclear plant engineers reduce speculation and gain reliable tools for developing mitigation strategies. Weber says the group intends to study other molten oxides using the same technique. The work was supported by the Department of Energy
The paper is “Molten uranium dioxide structure and dynamics,” Science (DOI: 10.1126/science.1259709).
Navrotsky’s Perspectives article is “Taking the measure of molten uranium oxide,” Science (DOI 10.1126/science.aaa0163).
Coincidentally, Navrotsky just published a paper in the November Journal of the American Ceramic Society on high-temperature characterization, “Progress and new directions in calorimetry: A 2014 perspective.” The article reviews technical evolution of calorimetric techniques for oxide melts and is free.
Author
Eileen De Guire
CTT Categories
- Basic Science
- Energy
- Environment
- Modeling & Simulation