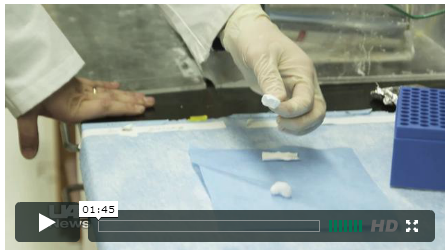
A lot of the basics of electrospinning have been figured out and it is relatively easy to use a desktop apparatus to spin mats of a particular material or even functionally graded materials, so a lot the recent emphasis on electrospinning has been devoted to scaling up these systems for mass production. However, a group of investigators the University of Alabama at Birmingham’s School of Engineering have come up with an ingenious method of using electrospinning to build a superior three-dimensional scaffold of nanofibers that, it is hoped, will provide a boost to tissue engineering.
The problem the group was trying to solve when they made their discovery was that the typical electrospun mat doesn’t do so well serving as a framework for facilitating cell and tissue growth. The fibers’ mass (along with remaining solvents) tended to flatten these mats, making them less than ideal for penetration by growing cells. Nanoscaffolds support the adhesion, growth and function of various cell types as they mature into specific tissues such as tendons, muscles and bones during tissue engineering. Yet, the traditional industry method for electrospinning creates densely packed sheet-like structures that prevent cells from penetrating the nanoscaffolds. In a paper in Biomaterials titled ”Cell infiltration and growth in a low density, uncompressed three-dimensional electrospun nanofibrous scaffold,” (doi:10.1016/j.biomaterials.2010.10.056), UAB’s Ho-Wook Jun and others in his group describe how they created their method to build a three-dimensional “cotton ball” of electrospun polycaprolactone that has the more desirable low density structure. The key, according to Jun and the other authors is to used the inside of a grounded, curved dish instead of the typical flat-plate collector coupled with an array of electrospinning syringes. Aptly, they have cleverly named this method “FLUF,” their acronym for Focused, Low density, Uncompressed nanoFiber mesh scaffold. In a UAB news release, Bryan A. Blakeney, a recent graduate student and one of the authors is quoted as saying, “Our three-dimensional electrospun nanoscaffolds better mimic nature and encourage cells to live longer and generate more viable, or functional, tissues. Think of it like a bowl of rocks: You pour a liquid into the bowl, and the liquid fills all the large gaps between the rocks. But with our 3D nanoscaffold, our rocks, or the fibers that constitute the scaffold, are the size of sand particles. We’ve separated the grains of sand to create gaps, and the cells fill and penetrate the gaps between the sand grains to form tissues.” In the paper, the group says they tested the performance of the FLUF ePCL material and report, “Cells seeded on the cotton ball-like scaffold infiltrated into the scaffold after seven days of growth, compared to no penetrating growth for the traditional electrospun scaffold. Quantitative analysis showed approximately a 40 percent higher growth rate for cells on the cotton ball-like scaffold over a seven-day period, possibly due to the increased space for in-growth within the three-dimensional scaffolds.” For me, the payoff seems to be that the FLUF method isn’t limit to ePCL and should be suitable to those investigating the use of inorganic fibers for tissue engineering, too. In addition to Jun and Blakeney, the authors include Ajay Tambralli, Joel M. Anderson, Adinarayana Andukuri, Dong-Jin Lim and Derrick R. Dean. |