[Image above] Could Jenga help students understand how lithium-ion batteries work? Credit: Driscoll et al., Journal of Chemical Education (CC BY 4.0)
Crack open almost any electronic gadget and you’ll likely find a lithium-ion battery inside.
Li-ion batteries are the foundation for most of today’s advanced electronics, from portable devices (like smartphones) to electric vehicles and renewable energy storage. No wonder three scientists who helped develop the technology were awarded the Nobel Prize in Chemistry last year.
Because Li-ion batteries play such a big role in our everyday lives, this topic is important for students to understand. But how does one easily and clearly communicate Li-ion battery science to students who may have very little science background? In a new paper, a team of researchers from the University of Birmingham’s School of Chemistry offer a unique and fun approach.
Jenga to the rescue: Modified tower block game aids understanding of rechargeable Li-ion batteries
In the paper’s introduction, the researchers explain their study grew from a desire to overcome some limitations inherent in current battery demonstrations.
“The most commonly used activity [to explain battery operation] employs lemon-/potato-electrolyte batteries, which are useful for introducing the concept of electrode potentials, electrical circuits, and a non-rechargeable battery,” they write. “Although these are good hands-on activities to introduce key aspects of batteries, these demonstrations cannot explain how rechargeable batteries work and, additionally, often lead to a misconception that the lemon or the potato is the powerhouse behind the circuit.”
The researchers decided to explore using tower block games like Jenga to explain Li-ion battery operation because “The archetypal Li-ion battery with electrode materials LiCoO2–graphite is a layered system, and hence, the stacking of blocks in a normal tower block game makes this traditional set ideal for customization into the appearance of this battery setup,” they write.
The researchers purchased two tower block sets and designated one set to be the lithium cobalt oxide cathode and the other as the graphite anode. Then, they painted the stacked blocks to indicate different components: cobalt oxide layers were painted purple with red spheres; graphite layers were painted gradient gray; lithium ions were painted as blue dots; blank spaces (indicating where lithium ions could move) were painted white; and aluminum and copper current collectors were painted gray and orange, respectively.
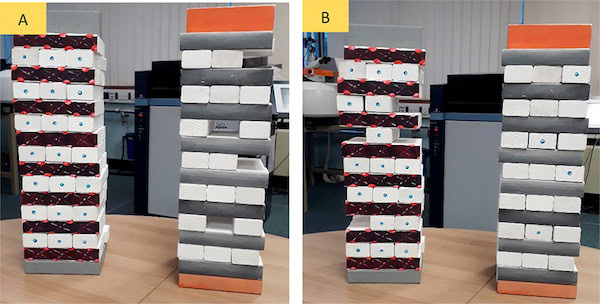
Painted tower block game sets with oxide electrode (left) and graphite electrode (right). (A) The graphite electrode shows vacancies where the lithium blocks can be inserted, as shown in part B on charging from the oxide electrode. Credit: Driscoll et al., Journal of Chemical Education (CC BY 4.0)
Using this setup, the researchers demonstrated three important aspects of Li-ion battery operation.
Intercalation
Intercalation, or the reversible insertion of a molecule into materials with layered structures, is the process that takes place when charging a battery.
The researchers demonstrated the intercalation process by removing the blue dot Li-ion blocks from between the cobalt oxide layers and inserting them between graphite layers in the second tower. By reversing the motion, they showed the processes that occur during discharging.
Overcharging
In real Li-ion batteries, “overcharging (removing more than x = 0.5 Li from Li1–xCoO2) can result in breakdown of the material, as CoO2 is an unstable intermediate and there is a resultant oxygen release, which can oxidize the electrolyte and result in the danger of a battery fire,” the researchers explain.
The overcharging concept is mirrored in the tower block sets by the fact three blue dot Li-ion blocks cannot be removed from one row—otherwise, the tower would collapse, similar to a real battery potentially catching fire.
Fading capacity
One reason a battery’s capacity reduces over time is because continued removal and insertion of lithium ions causes distortions in the electrode structure.
The researchers note this distortion can be seen in the block tower as well after multiple rounds of removing and reinserting the blue dot Li-ion blocks.
In addition, fast collapse of a battery that occurs when it is charged too quickly can be demonstrated by removing the blue dot Li-ion blocks quickly without care.
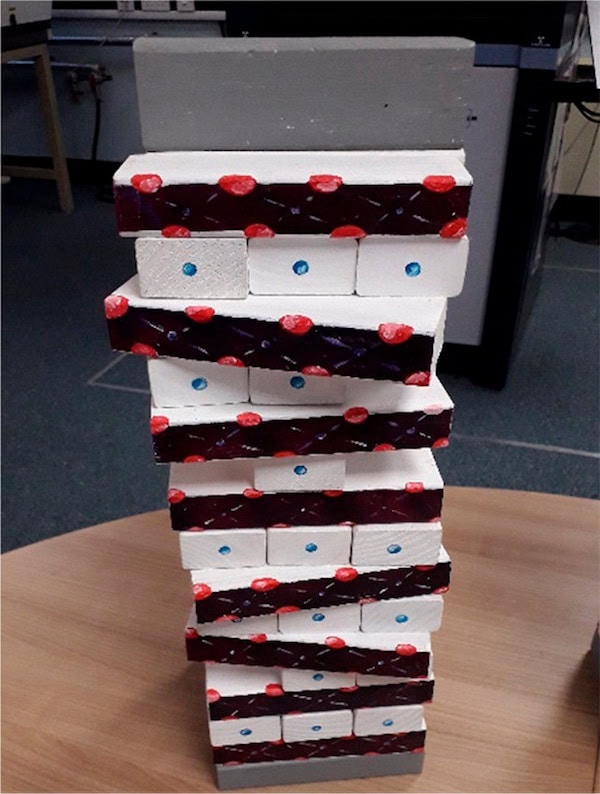
Oxide electrode tower block set shows displaced cobalt oxide layers due to (de)intercalation of the lithium during cycling. Credit: Driscoll et al., Journal of Chemical Education (CC BY 4.0)
The researchers trialed the tower block battery at multiple schools and museums over the past year with positive feedback, which is detailed in the paper. In the future, they plan to make the activity more accessible to all students, particularly students who are blind or partially sighted, by adding texture to the blocks (a preliminary example of which is illustrated below).
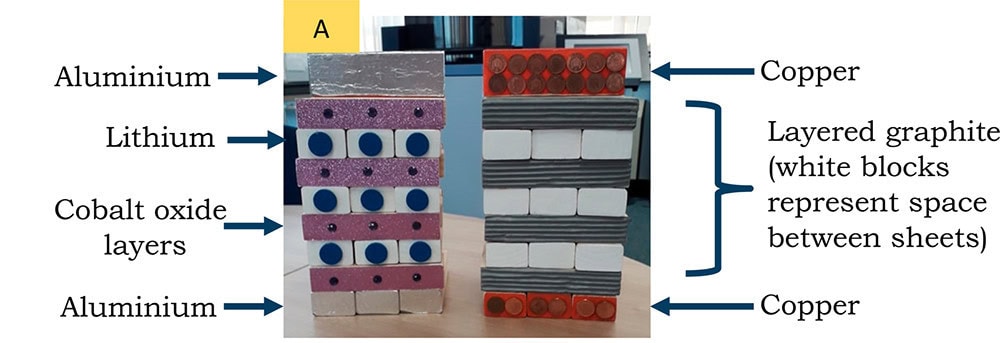
Example of a tactile block tower setup in which wood, plastic, cardboard, and other materials are used to make the blocks distinguishable from each other. Credit: Driscoll et al., Journal of Chemical Education (CC BY 4.0)
The open-access paper, published in Journal of Chemical Education, is “The building blocks of battery technology: Using modified tower block game sets to explain and aid the understanding of rechargeable Li-ion batteries” (DOI: 10.1021/acs.jchemed.0c00282).
Interested in more fun science demos?
Check out ACerS new “Communicating Science” webpage, which features tips and examples of effective science communication methods.
Author
Lisa McDonald
CTT Categories
- Education
- Energy