[Image above] Photocatalytic water splitting offers a clean way to produce hydrogen—if suitable materials for photocatalysts are found. Credit: Royal Society of Chemistry, YouTube
Renewables are not the only low-carbon technology seeing a ton of growth recently.
“Hydrogen has never enjoyed so much international and cross-sectoral interest, even in the face of impressive recent progress in other low-carbon energy technologies, such as batteries and renewables,” the International Energy Agency (IEA) says in their recently-released report, “The Future of Hydrogen,” as reported by Bloomberg. In fact, since 1975, demand for hydrogen has grown more than threefold around the world!
In theory, hydrogen is a desirable alternative to fossil fuels because it gives off water when burned rather than deleterious greenhouse gases such as carbon dioxide and methane. In practice, however, hydrogen is not quite so clean.
Hydrogen fuel is produced by extracting hydrogen from fossil fuels, biomass, and/or water. When extracted by water electrolysis, hydrogen production is a fairly clean process. Unfortunately, as the IEA webpage summarizing the report explains, less than 0.1% of global dedicated hydrogen production comes from that method. Instead, “Natural gas is currently the primary source of hydrogen production, accounting for around three quarters … [and] gas is followed by coal.”
So, even though burning hydrogen fuel is clean, producing hydrogen currently relies on carbon-intensive methods.
Yet with declining costs for renewable electricity—particularly solar and wind—interest is growing in electrolytic water-splitting methods. And another method for water splitting is gaining attention as well—photocatalysts.
While electrolytic water splitting involves passing an electrical current through water to drive a chemical reaction, photocatalytic water splitting is a type of artificial photosynthesis that places photocatalysts (light-absorbing materials) in water to drive water oxidation. Research on photocatalytic water splitting focuses on finding stable photocatalysts that consistently demonstrate higher solar-to-hydrogen conversion efficiency.
Tantalum nitride (Ta3N5) is one of the few visible light-absorbing photocatalysts capable of overall water splitting (OWS), a process in which hydrogen and oxygen are produced simultaneously in a 2:1 stoichiometric ratio. When Ta3N5 absorbs light, electron-hole pairs are generated and these charge carriers migrate toward the Ta3N5 surface to then react with water.
However, achieving OWS in Ta3N5 remains a challenge.
Researchers have observed that efficient generation of hydrogen is fundamentally less pronounced than oxygen in Ta3N5. The reason for this difference is unclear, but researchers believe it may be due to how Ta3N5 is prepared.
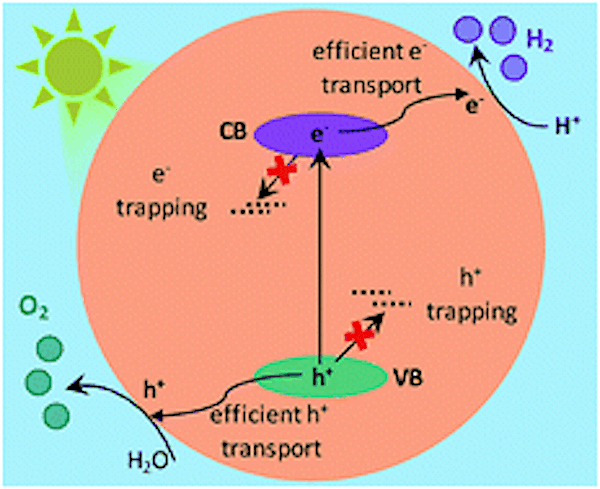
When Ta3N5 absorbs light, electron-hole pairs are generated and migrate toward the surface. Some charge carriers become trapped along the way in structural and electronic defects. Credit: Murthy et al., Chemical Science 2019, 10, 5353-5362 (Published by The Royal Society of Chemistry, CC BY 3.0)
Ta3N5 is commonly prepared by nitridation reaction of tantalum precursors such as tantalum foil or tantalum oxide (Ta2O5). Researchers assume tantalum precursors and nitridation times likely play a role in creating structural and electronic defects in Ta3N5, which affect the ability of charge carriers to move to the surface and thus reduces the photocatalyst’s water-splitting ability.
In a recent open-access study published in Chemical Science, researchers from multiple universities and institutes in Japan used transient absorption spectroscopy to investigate how nitridation time affects OWS ability in Ta3N5 created from either Ta2O5 or potassium tantalate (KTaO3).
The researchers found OWS efficiency was highest in Ta3N5 prepared by 0.25-hour nitridation of KTaO3; conversely, 0.25-hour nitridation of Ta2O5 formed neither the Ta3N5 phase nor showed OWS. In fact, “For Ta3N5 prepared by the nitridation of Ta2O5, due to electron trapping within the bulk, efficient migration of electrons towards the surface cannot be anticipated,” the researchers conclude in their paper.
In addition to observing how choice of tantalum precursor affects OWS ability, the researchers found the amount of near-infrared absorption increased when nitridation time increased from 0.25 to 10 hours, indicating longer nitridation time increases the presence of defect states within the bandgap of Ta3N5, thus lowering OWS efficiency.
The researchers end their study with a suggestion on how to further increase OWS efficiency in Ta3N5 created from KTaO3. “Upon 0.25-hour nitridation of KTaO3, the actual amount of Ta3N5 phase formed on KTaO3 is [about] 2%,” they explain. Multiple nitridation could potentially increase the effective density of Ta3N5 on KTaO3, boosting OWS efficiency.
Interested in research on photocatalysts? A CTT article I wrote in February shows how photocatalysts can split carbon dioxide to produce alternative fuels as well!
The open-access paper, published in Chemical Science, is “Origin of the overall water splitting activity of Ta3N5 revealed by ultrafast transient absorption spectroscopy” (DOI: 10.1039/c9sc00217k).
Author
Lisa McDonald
CTT Categories
- Energy
- Environment