[Image above] Secondary electron micrograph showing a Cr2AlC film after nano-impact testing. This MAX phase system is being considered for use in next-generation nuclear power technologies. Credit: Tunes et al., Science Advances (CC BY-NC 4.0)
Here on CTT, we cover quite a bit of research on MXenes, which are a family of 2D transition metal carbides, carbonitrides, and nitrides that are made by selectively etching MAX phases. However, we don’t always cover the MAX phases themselves, even though these materials have seen a considerable resurgence for many applications.
MAX phase is the term for layered ceramic materials with the general formula Mn+1AXn, where M is a transition metal (toward the left-hand side of the periodic table), A is a metalloid (edging into the left-hand transition metals and heavy metals), and X is carbon or nitrogen. Researchers have studied over 150 compositions with this general formula, according to a recent review article in Journal of the American Ceramic Society (JACerS).
With their unique layered structures, MAX phases offer a beneficial mixture of properties, such as the machinability and electrical conductivity of metals with the high temperature and corrosion properties of ceramics. In addition, MAX phases can be synthesized through a variety of methods for structural and coating applications.
The radiation tolerance of MAX phases is of particular interest to researchers because it makes these materials ideal candidates for use in next-generation nuclear power technologies. Studies that exposed various MAX compositions to low and high doses of high-energy ions found that the collisions result in reorganization of the crystal structure, often completely disrupting long-range order and converting the material to amorphous structures. However, mobility of the atoms within the MAX phases enables recovery of the structure through annealing at moderate temperatures.
Many irradiation studies have focused on the titanium-based MAX phases due to their ability to retain crystallinity at high radiation doses. “However, instabilities in Ti-based MAX phases under proton irradiation recently concluded that these materials may have limited applicability in high-temperature reactors,” researchers write in a recent open-access paper.
Ternary carbides within the Cr–Al–C system are an alternative MAX phase that offers a couple important advantages: It can be produced at relatively low temperatures, and the material can be first deposited in amorphous form then crystallized in air without any deterioration. But to date, there are limited radiation studies on this system.
Fortunately for us, two recent papers do look at irradiation of this MAX phase system. The first one, published September 2020 in JACerS, is led by researchers at Fudan University in China; it investigated irradiation with helium ions. The second one, published March 2021 in Science Advances, is by researchers in Austria and the United Kingdom; it investigated irradiation with xenon ions.
Both papers synthesized Cr2AlC films using magnetron sputtering, a physical vapor deposition technique which can be employed on an industrial scale. However, the sputtering conditions of the two studies were quite different.
(For those unfamiliar with physical vapor deposition, inert ions (typically argon) are energetically directed at solid targets formed from the elements to be deposited. The inert ions dislodge desired ions from the target, which are attracted to and ultimately deposit on an oppositely charged substrate/support.)
The authors of the JACerS article deposited the film on alumina substrates at 600°C, using a combined Cr/Al target in a low-pressure acetylene/argon environment. (Acetylene is the carbon source for this study.) The resulting Cr2AlC is highly crystalline.
The authors then irradiated the samples with 100 keV helium ions, with the substrate kept at ambient temperature. The heating due to the irradiation resulted in the sample equilibrating at about 70°C. During the dosing process, the Cr2AlC structure was disrupted, though the solid solution (Al, Cr)Cx was formed with the crystal structure shown below. The authors found little evidence of an amorphous phase. The implantation of the helium ions elongated the c-axis of the crystals relative to the virgin (undoped) material.
Upon annealing, the material returned to the original crystal structure, partially at 430°C and fully by 600°C. Further tests showed slight migration of the implanted helium toward the surface at these temperatures. When annealed at 750°C, the helium migrates, forming surface bubbles and surface holes and partially delaminating the film from the alumina substrate.
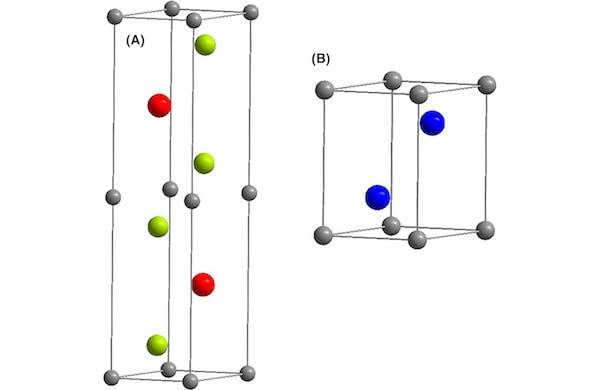
Crystal structures of (A) as-synthesized Cr2AlC and (B) the (Cr,Al)Cx solid solution formed by irradiation. The green, red, and gray balls represent chromium, aluminum, and carbon atoms, respectively. The blue balls represent the cation site randomly occupied by chromium or aluminum atoms. Credit: Wang et al., Journal of the American Ceramic Society
In contrast to the JACerS article, the authors of the Science Advances paper used noticeably different deposition parameters. Their goal, more than just investigating effects of irradiation, was to investigate how the material would behave under irradiation if it was not fully crystalline so as to better understand the fundamental processes behind its radiation tolerance. As such, the authors “deviate from the pure MAX phase concept by synthesizing a dual-phase material with the Cr2AlC MAX phase as matrix and an amorphous phase of the same local chemistry, as the secondary phase.”
First, synthesis was performed at 648 K (375°C), which is below even the annealing temperature of 430°C used in the JACerS paper. The authors used three targets (Cr, Al, C) in an inert low-pressure argon atmosphere. The elements were deposited in stepwise layered fashion with the substrates rotating past the targets in a single chamber.
The film that resulted from this synthesis had both nanocrystalline MAX phases and amorphous grain boundaries of the same composition. The X-ray diffraction pattern shows the expected crystal pattern peaks along with a broad amorphous response at lower angles.
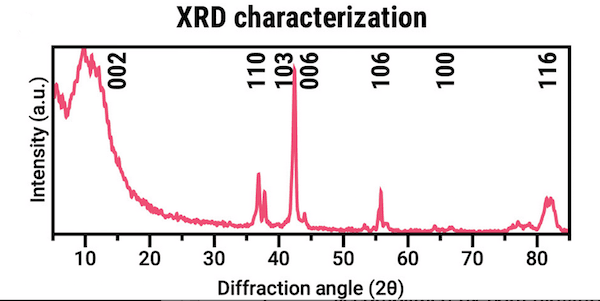
The XRD characterization (above) confirmed the TEM and STEM-EDX results that showed a low degree of crystallinity in the investigated material, which indicates the presence of an amorphous phase. Credit: Tunes et al., Science Advances (CC BY-NC 4.0)
To irradiate the film, the authors implanted much larger and higher energy ions (300 keV xenon ions) at 623 K (375°C), in addition to higher dosing (40 displacements per atom). As with the previous study, the irradiation did not result in large-scale creation of amorphous regions.
Instead, while there was some implantation in the crystalline phase, the bulk of the xenon implanted into the amorphous grain boundaries.
Post-irradiation transmission electron microscopy shows thickening of the grain boundaries due to larger xenon bubbles, which is accompanied by both radiation induced segregation of chromium at the grain boundaries and chromium clustering at regions close to the edges of the bubbles.
The observation that larger xenon bubbles accumulated mainly in the grain boundaries is a substantial finding—it indicates that nanocrystalline grain sizes are effective sinks for radiation induced defects, which also helps to prevent full amorphization of the MAX phase. With minimal effects on the crystallite structure and lattice parameters, this dual microstructure should retain structural integrity after irradiation to a larger degree.
The authors discuss various paths forward for extending the investigation and application of their work. For example, they plan to conduct more studies on semicrystalline and amorphous nanolayered ceramics within the context of radiation damage effects, and they will consider compatibility with different substrates, metallic claddings, and the coolant material, including corrosion studies.
In conclusion, while both papers show that Cr2AlC MAX phases synthesized via magnetron sputtering are resistant to damage from high-energy irradiation, the differences in structure and damage mitigation mechanisms are intriguing. It would not surprise me to see optimization of production parameters leading to use of this material in next-generation nuclear power technologies.
Of course, there are many other MAX phases to be explored beyond the titanium and Cr–Al–C systems. A recent study by Texas A&M researchers describes a new computational tool to evaluate the suitability of different MAX phases for high-temperature applications. Learn more in the Texas A&M press release, and look for in-depth coverage this Friday on CTT! (Update 04/23/2021 – See the Friday article here.)
The 2020 paper, published in Journal of the American Ceramic Society, is “Annealing effects on the structure and hardness of helium‐irradiated Cr2AlC thin films” (DOI: 10.1111/jace.17469).
The 2021 open-access paper, published in Science Advances, is “Deviating from the pure MAX phase concept: Radiation-tolerant nanostructured dual-phase Cr2AlC” (DOI: 10.1126/sciadv.abf6771).
Author
Jonathon Foreman
CTT Categories
- Energy