[Image above] Ferroelectric crystals that were treated with either AC (left) or DC (right) electric fields. Credit: Bo Wang, The Pennsylvania State University
If you are a regular follower of Ceramic Tech Today, you know this year’s January/February issue of the Bulletin was all about ferroelectricity.
Ferroelectricity is a characteristic of certain materials exhibiting spontaneous electric polarization that can be reversed by the application of an external electric field. Ferroelectric materials were first reported on in 1920, which means this year is the 100th anniversary of their discovery.
One big application of ferroelectric materials is piezoelectric transducers, or devices that convert pressure or mechanical stress into an electrical charge. In particular, perovskite ferroelectric ceramics and crystals, such as lead magnesium niobate-lead titanate (PMN-PT), are commonly used because they possess a very high piezoelectric coefficient d33 and an excellent electromechanical coupling factor k33—i.e., they convert mechanical energy into electrical energy very well (they have high piezoelectricity).
However, a tradeoff of this high piezoelectricity is these perovskite ferroelectrics are usually opaque in the visible-light spectrum. The opacity is caused by light scattering and reflection from their ferroelectric domain walls, i.e., boundaries between separate regions of uniform polarization.
A material with both high piezoelectricity and high transparency would be beneficial in a wide range of applications, including medical imaging and self-energy-harvesting touch screens. Unfortunately, possible approaches to increasing transparency, such as creating a single-domain state (reducing the number of domain walls) or breaking domains into polar nanoregions (increasing the number of domain walls), often cause piezoelectricity to decrease.
Though these particular methods of changing the number of domain walls to achieve high transparency and piezoelectricity were unsuccessful, that does not mean changing the number of domain walls is a bad approach. Rather, a better question to ask is which domain walls are being changed, not how many.
A recent study led by researchers from The Pennsylvania State University and Xi’an Jiaotong University in China now shows that different domain walls account for a very (literally) transparent difference in properties.
The discovery comes as a result of a different question asked by the researchers—why do cycles of alternating current (AC) fields affect piezoelectric crystals differently than direct current (DC) fields?
“There have been reports that the use of AC fields could significantly improve the piezoelectric responses—for example by 20% to 40%—over DC fields,” Long-Qing Chen, Hamer Professor of Materials Science and Engineering, professor of engineering science and mechanics, and professor of mathematics at Penn State, says in a Penn State press release.
He explains that improvements are traditionally attributed to smaller domain sizes resulting from cycles of AC fields. However, about a year ago when Chen’s group started modeling internal domain structures of perovskite ferroelectrics, “Our computer simulations and theoretical calculations did show an improved piezoelectric response, but our simulations also demonstrated that the ferroelectric domain sizes actually got bigger during AC cycles rather than smaller as reported in the literature,” Chen says.
To investigate why their simulations contradicted previous reports, they reached out to colleagues at Xi’an Jiaotong University for help. The Xi’an Jiaotong researchers grew their own PMN-PT crystals and carefully examined the domain configurations under different AC cycling conditions—and confirmed what the Penn State researchers saw in their simulations.
In the paper on their research, the researchers explain what is happening to the domain structure.
“Three types of domain wall are present in the unpoled rhombohedral crystal: 71°, 109° and 180° domain walls,” they write. “Under a d.c. electric field along the [001] direction … only 71° and 109° domain walls survive, whereas the 180° domain walls are eliminated.”
In contrast, “Our phase-field simulations demonstrate that the application of an a.c. electric field effectively reduces the number of 71° domain walls, with only two 71° domain walls left in each lamina after a.c. poling, leading to a much larger domain size within each lamina,” they explain.
Essentially, domain walls in PMN-PT crystals exist in three natural orientations: 71°, 109°, and 180°. The 71° domain walls can scatter light, while 109° domain walls do not. Cycling an AC field reduces the number of 71° domain walls in the crystal, meaning domain sizes are larger and light can more easily pass through the crystal—leading to a transparent material.
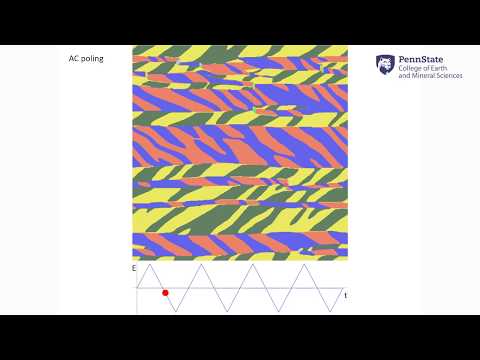
Video illustrating how cycling AC fields removes 71° domain walls. The start of the video shows an unpoled sample; up to 3 seconds illustrates the effects of a DC field; after 3 seconds illustrates the domain changes following AC field cycling. 71° domain walls are the diagonal walls within alternating colored strips. Credit: Penn State University, YouTube
Additionally, the presence of 71° domain walls causes deviation of the polarization from the ideal direction. With the 71° domain walls removed, polarization can occur in the ideal direction—resulting in the crystal’s piezoelectricity increasing in addition to the transparency.
The researchers note these results are unique to PMN-PT crystals and enhanced piezoelectricity with increasing domain size is not universal to all ferroelectric crystals.
“It is expected that the piezoelectricity of ferroelectrics is also related to the symmetry of the ferroelectric phase and domain configurations,” they write. “For example, in tetragonal BaTiO3 and high-temperature-poled PZN-PT crystals, where domain configurations are very different from PMN-28PT, the domain-size dependence of piezoelectricity shows an opposite trend to that observed here.”
Nonetheless, “This work presents a paradigm for achieving high transparency and piezoelectricity by ferroelectric domain engineering, and we expect the transparent ferroelectric crystals reported here to provide a route to a wide range of hybrid device applications,” they conclude.
The researchers from Penn State and Xi’an Jiaotong jointly filed for a U.S. patent on this work.
The paper, published in Nature, is “Transparent ferroelectric crystals with ultrahigh piezoelectricity” (DOI: 10.1038/s41586-019-1891-y).
Author
Lisa McDonald
CTT Categories
- Electronics
- Modeling & Simulation
Spotlight Categories
- Member Highlights