[Image above] Screenshot from a video showing the disintegration of a ceramic as it attempts to undergo a tetragonal-to-monoclinic phase transformation. Credit: Gu et al., Nature (CC BY 4.0)
As the holiday season gets into full swing, I know full well how easy it is to let exercise routines slip. Unfortunately for us, we require a lot more work to regain our pre-vacation bods than it does for shape memory materials to regain their original form.
Shape memory materials are materials that can recover their initial shape under the influence of external stimuli, such as temperature, light, moisture, acidity, or electricity. Certain metal alloys were the first materials identified to exhibit the shape memory effect, and researchers have identified a variety of shape memory polymers and polymer composites that exhibit the effect as well.
The shape memory effect is based on the material undergoing a phase change when exposed to external stimuli. In the case of shape memory polymers, the change between a hard phase to soft phase can be achieved through a variety of physical means due to the intrinsic elasticity of polymeric networks. For shape memory alloys, the effect stems from the material having two stable crystal structures: a high temperature-favored austenitic phase and a low temperature-favored (and “yieldable”) martensitic phase.
(Learn more about the phase change process in this article.)
When shape memory alloys undergo transformation from one solid phase to another, they can experience a stressed transition layer that separates the twinned martensite phase from the austenite phase. This stress affects the alloys’ ability to reverse the transformation.
To improve reversibility of the solid–solid phase transformation, researchers try to improve the geometric compatibility of the alloy. In other words, they carefully tune the lattice parameters in the alloy to decrease the elastic energy in the transition layer, and thereby improve the alloy’s ability to reversibly transform between the two solid phases.
Like alloys, ceramics consist of a crystalline structure and have different stable solid phases. This similarity between alloys and ceramics, particularly zirconia-based ceramics, has led to some success in developing shape memory ceramics by applying the same general strategies used for alloys. For example, a few recent studies improved the phase transformation of various oxide systems by improving the geometric compatibility.
Based on these previous successes, an international team of researchers led by the University of Minnesota Twin Cities and Kiel University in Germany recently conducted a systematic search to identify more ceramics that exhibit reversible phase transformations and shape memory behavior based on improving geometric compatibility. However, as they explain in a new paper published in Nature, they stumbled upon a seemingly paradoxical example instead.
For their study, the researchers looked at the ceramic system (Zr/Hf)O2(YNb)O4. This system shows the same phase transformations as bulk ZrO2—a first-order monoclinic-to-tetragonal phase transformation at 1,170°C and a tetragonal-to-cubic transformation at 2,370°C. (Only the first transformation is relevant to this study.)
After tuning the lattice parameters to improve the geometric compatability, the researchers tried cooling the ceramic through its tetragonal-to-monoclinic phase transformation. However, instead of transforming, the polycrystal slowly and steadily fell apart at its grain boundaries (a process the researchers termed weeping) or even explosively disintegrated.
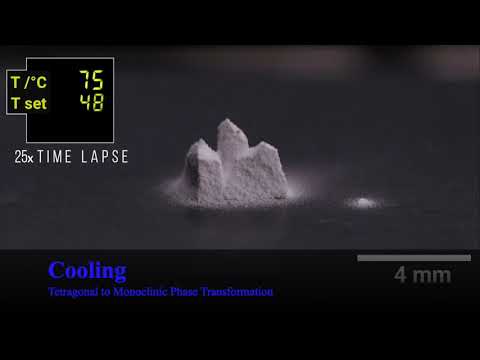
A sample with a high zirconium/hafnium ratio that exhibits weeping (that is, steady falling apart at the grain boundaries) on cooling to the transformation temperature. Credit: Jascha Rohmer, Kiel University
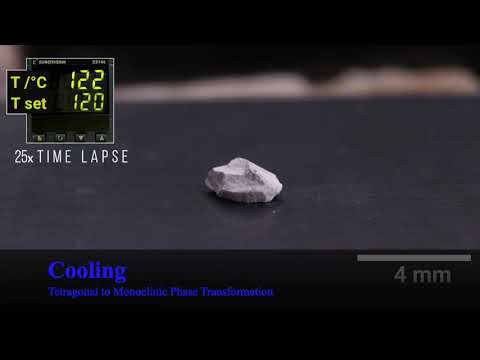
A sample with a high zirconium/hafnium ratio exhibiting explosive behavior on cooling to the transformation temperature. Credit: Jascha Rohmer, Kiel University
What mechanism could have caused this unexpected result? The researchers suggest the behavior is due to the (Zr/Hf)O2(YNb)O4 system having multiple lattice correspondences, i.e., multiple possibilities for which atoms go where during transformation.
The researchers tuned the lattice parameters based on one of these correspondences, which should have made it easier for the material to undergo transformation. However, it instead appeared to have introduced strain elsewhere in the structure, leading to the disintegration.
The researchers then tried modifying the structure based on an equidistance condition, which takes into account multiple correspondences. This time, the ceramic did demonstrate a reversible transformation.
“While these behaviours seem at first to contradict accepted principles, in fact they serve to widen the set of theory-driven tools applicable in the search for reversible transformations in ceramics,” the researchers write. “Our work also highlights the subtle interplay of multiple transformation correspondences.”
The researchers conclude that their work highlights the need for a general theory of compatibility that includes both phase boundaries and grain boundaries, and a full-atomic-resolution experimental study of grain boundaries in transforming ceramics.
The paper, published in Nature, is “Exploding and weeping ceramics” (DOI: 10.1038/s41586-021-03975-5).
Author
Lisa McDonald
CTT Categories
- Basic Science