[Image above] The Volvo XC90 line of premium SUVs is known for its heavy use of boron steel throughout the vehicle’s structure to improve safety in a crash. Credit: dmytrok, Flickr (CC BY-ND 2.0)
When we talk about construction materials on CTT, we often focus on concrete—it is the most used building material (and second most consumed material) in the world, after all. But there is another widely used building material that also owes its existence to ceramics—steel.
Steel is an alloy of iron that contains small amounts of carbon to improve its strength and fracture resistance. Early blacksmiths discovered steel accidentally when they observed that iron became harder and stronger after combining with the carbon in a charcoal-fueled furnace.
All steels are made using iron and carbon, but manufacturers will add additional elements to this base formula to achieve steels with different properties. For example, adding chromium results in a steel alloy called “stainless” for its ability to resist rust.
Boron is another material sometimes added to steel to enhance the material’s hardenability, strength, and wear resistance. The first systematic study of boron additions to steel took place over a century ago, in 1907. Today, boron steels are used in the automotive industry as strengthening elements, such as around the door frames and in reclining seats, and in the nuclear industry as shielding due to boron’s high neutron absorption capabilities.
Boron typically makes up less than 1 wt.% of the steel alloy. When higher amounts of boron are added, the boron segregates from the steel and settles in the grain boundaries, which lowers the alloy’s hardenability, reduces toughness, induces embrittlement, and can cause problems in welding.
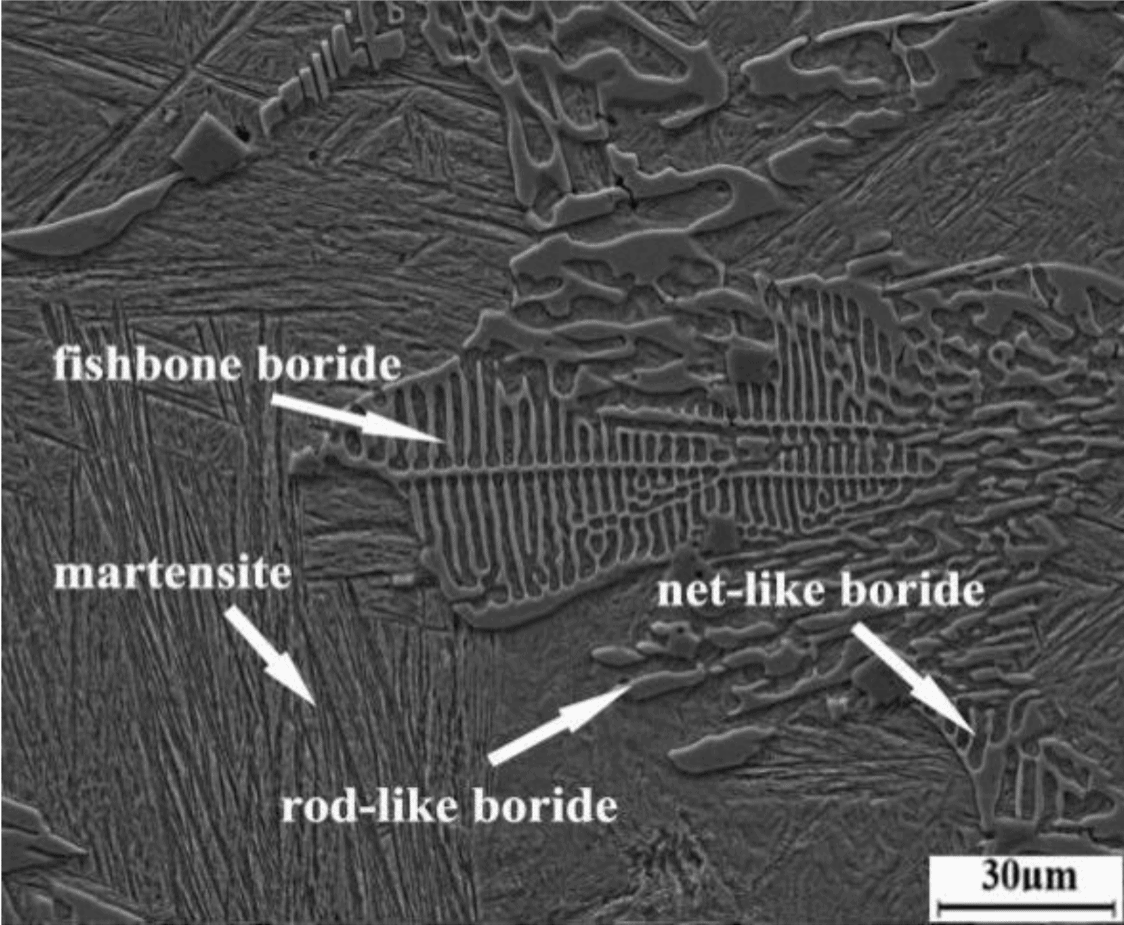
Scanning electron micrograph showing different morphologies of boron segregation in high boron steels. The segregation seriously damages continuation of the matrix, resulting in reduced toughness and embrittlement. Credit: Li et al., Materials (CC BY 4.0)
To improve the toughness of high boron steels, researchers have focused on approaches that disrupt the segregation and formation of different borides, such as alloying and high-temperature heat treatments. While these methods improve boride morphology, “[they] have a very limited effect on enhancing the toughness of the matrix,” researchers write in a recent open-access paper.
The researchers come from Wuhan University of Science and Technology in China, in addition to colleagues from Valin ArcelorMittal Automotive Steel Co. Ltd. (China) and University of New South Wales (Australia). In their paper, they wanted to improve the alloy’s matrix directly rather than just mitigating boride formation. To do so, they used a novel process called quenching and partitioning (Q&P).
Q&P allows for a certain iron crystal structure called austenite to be retained in the matrix by stabilizing it with carbon that diffuses from a different crystal structure called martensite. There are three main steps to this process:
- The steel is austenitized, i.e., heated to a temperature at which it changes crystal structure from ferrite to austenite.
- The steel is quenched to allow partial transformation to the martensite form (leaving some austenite untransformed).
- The steel is annealed either at or above the initial quench temperature to allow for partitioning to take place, i.e., diffusion of carbon from the martensite to retained austenite.
The researchers performed a Q&P heat treatment process using an electric furnace and salt baths to create high boron steel with 1.6 wt.% boron. They also cast a sample of the high boron steel without Q&P treatment to compare.
The researchers observed a few key structural differences between the as-cast and Q&P-treated samples. Specifically, the borides in the Q&P-treated sample were partially broken and spheroidized, thus disrupting the net-like and rod-like morphologies seen in the as-cast sample. In addition, increased quenching times allowed the retained austenite to distribute more uniformly and form as a film between the martensite areas, in contrast to the block morphology seen in the as-cast samples.
These differences in structure translated to differences in mechanical properties. The hardness of the Q&P-treated samples decreased as the amount of retained austenite increased, due to the austenite being softer than the martensite form. On the other hand, the toughness and wear resistance of the samples generally improved, a result attributed both to the change in boride morphology and also to the increase of retained austenite.
The table below shows hardness and impact toughness of samples treated at different quenching times; the amount of retained austenite increased with increased quenching times.
Quenching time (s) | Hardness (HRC) | Impact toughness (J·cm−2) |
30 | 61.8 | 4.4 |
60 | 58.0 | 5.6 |
90 | 56.1 | 7.5 |
120 | 55.3 | 6.3 |
Credit: Li et al., Materials (CC BY 4.0)
At excessively high fractions of retained austenite (obtained at quench times of 120 seconds), the matrix was unable to support the boride, leading it to fall off from the interface and resulting in many brittle fractures. Thus, to maintain good toughness and wear resistance, “the volume fraction of [retained austenite] should be controlled at about 16%,” the researchers write.
The open-access paper, published in Materials, is “The effect of quenching and partitioning (Q&P) heat treatment on the microstructure and mechanical properties of high boron steel” (DOI: 10.3390/ma14061556).
Author
Lisa McDonald
CTT Categories
- Basic Science