[Image above] Optical microscopy (left) and transmission electron microscopy (right) images of a glass composite embedded with LiNbO3. Researchers in China discovered this composite exhibits a unique nonlinear optical response that is rarely seen in bulk materials. Credit: Shifeng Zhou
With the rise of data-driven materials discovery and design, there is increasing demand on information technologies to gather ever larger amounts of high-resolution data and to process them in shorter amounts of time. Fortunately, there is a scientific discipline uniquely suited to addressing the former part of this need—the field of nonlinear optics.
Nonlinear optics refers to the study of a wide range of interesting physical phenomena based on nonlinear relationships. “Nonlinear” means there is not a direct or straight-line relationship between variables. In other words, nonlinear materials and processes exhibit responses that are disproportionate compared to the external force(s) triggering it.
For example, polymers are considered nonlinear materials because they exhibit a disproportionate stress–strain response—the materials yield rather than fracture when reaching a certain amount of stress. For obvious reasons, nonlinear materials can be quite useful in certain applications because they withstand demanding conditions that would cause other materials to fail.
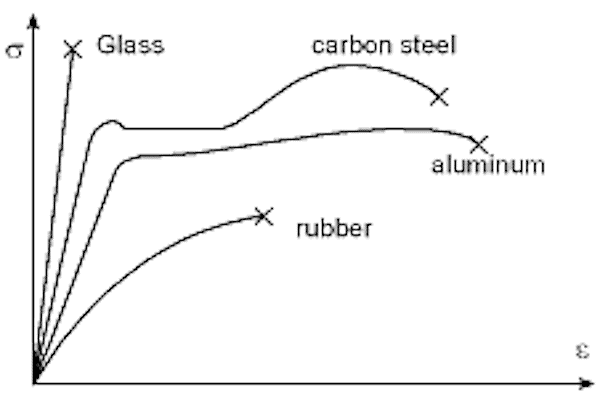
In the case of nonlinear optics, the nonlinear optical process called second-harmonic generation is highly studied because of its ability for high-resolution imaging. In this process, which is also known as frequency doubling, photons that interact with a nonlinear material will “combine” to form new photons with twice the frequency of the initial photons. This phenomenon allows for the creation of low-power and compact light sources that operate in wavelengths typically requiring more power and larger devices.
Since second-harmonic generation was identified in 1961, there now are numerous commercial devices based on this phenomenon available for purchase. These devices mainly use various crystals as the nonlinear material. However, researchers have started exploring the potential of using nanocrystal-in-glass composites instead.
Nanocrystal-in-glass composites are composed of tiny crystals embedded in a glassy matrix. These composites have emerged as an alternative to glasses and crystals in display applications because of some superior optical properties. For example, nanocrystal-in-glass composites containing high-quartz nanocrystals present zero thermal expansion over a wide temperature range of 0–50°C, which makes them ideal for use as optical mirror blanks in ultrastable space telescopes.
Some nanocrystal-in-glass composites, such as those embedded with LiNbO3 and LiNb0.5Ta0.5O3, are nonlinear materials and so have potential for use in second-harmonic generation devices. However, a recent study by researchers in China reveals these composites may have additional potential not previously realized.
The researchers are led by professors Shifeng Zhou and Huakang Yu from South China University of Technology. Originally, their goal was to study the nonlinear optical response of nanocrystal-in-glass composites with different domain structures in a counter-propagating laser scheme, i.e., a scheme where two laser beams enter the composite from opposite sides and interact. (Read this paper to learn why counter-propagating optical beam configurations often are used in nonlinear optics.)
While the nonlinear optical response in certain regions of the LiNbO3-embedded composites was as expected, the researchers surprisingly observed a local and brighter signal in an extreme region of the microstructure map—a signal that disappeared when either of the two laser beams were blocked.
“It is strange that this brighter signal would vanish while the background second-harmonic generation still existed when either individual pulse is blocked,” Zhou explains in an email.
They realized that the brighter signal must be due to a special nonlinear response called transverse second-harmonic generation. Transverse second-harmonic generation differs from standard second-harmonic generation in two significant ways.
- Phase matching mode: Standard second-harmonic photons are generated from the same pulse, whereas transverse second-harmonic photons are generated from oppositely propagating pulses.
- Generation condition: The only requirement for achieving standard second-harmonic generation is to fulfill the phase matching condition, i.e., minimize mismatch between interacting waves along the propagation direction. Transverse second-harmonic generation requires that, in addition to the phase matching condition, overlap of the oppositely propagating pulses in time and space must occur as well.
This difference in origin means that standard second-harmonic generation and transverse second-harmonic generation manifest differently as well. While the signal from standard second-harmonic generation can easily be observed at any position where the fundamental pulse passes, the signal from transverse second-harmonic generation can only be observed at the position where oppositely propagating pulses overlap.
The discovery of transverse second-harmonic generation in nanocrystal-in-glass composites is surprising because the phenomenon is typically observed in low-dimension nonlinear materials—seeing it in bulk nonlinear materials is rare. However, the fact it was discovered for the first time in a microstructured nonlinear photonic glass is exciting because of what it offers in terms of application.
“Standard second-harmonic generation is usually used for laser frequency conversion,” Zhou says. In contrast, because the transverse second-harmonic generation signal reflects the time domain information of the fundamental pulses, “the signal can be used for pulse group velocity and pulse width measurement of ultrashort optical pulses with femtosecond time scales.”
Pulse group velocity and pulse width are two values of critical importance in optical applications, so the ability to measure these values can improve the performance of numerous optical devices. For example, we can design fiber optic cables that are capable of carrying higher bandwidth signals over longer distances.
While this study focused on nanocrystal-in-glass composites embedded with LiNbO3, “It should be noted that the strategy should be universal to a wide category of nanoparticle-in-glass composites,” Zhou says. He adds that the researchers are now working on developing a novel fabrication strategy to further expand the nanocrystal-in-glass composites to more extreme regions, and they are exploring the composites’ potential for other unique properties and new practical applications.
The paper, published in Advanced Materials, is “Manipulating nonlinear optical response via domain control in nanocrystal-in-glass composites” (DOI: 10.1002/adma.202006482).
Author
Lisa McDonald
CTT Categories
- Basic Science
- Optics