[Image above] Materials discovery is a lot like world exploration—both can be time-consuming and risky endeavors. But maps can help guide explorers to more fruitful paths. Credit: Pixabay
“The smallest possible size of electronic component that can be crammed onto silicon computer chips will soon be reached,” says previous BBC news online science editor David Whitehouse in a BBC article—from 1999.
Twenty years later, people are still talking about the “imminent” demise of silicon. The difference between the 1990s and now, though, is that several technologies have matured enough to begin posing a threat to silicon’s dominance.
One material group that is the basis for several such technologies is nitrides. Nitrides refer to compounds of nitrogen in which nitrogen has a formal oxidation state of −3. The nitrogen anion N3− provides nitrides with unique electronic and bonding characteristics that give nitrides useful optoelectronic and defect-tolerance properties.
Though certain nitrides such as gallium nitride and boron nitride have received quite a bit of attention from the electronics sector, nitrides as a materials group remain vastly unexplored. Just take a look at the Inorganic Crystal Structure Database—while there are more than 4,000 ternary metal oxides included in the database, there are fewer than 400 unique ternary metal nitrides.
The reason there are so few known nitrides is due largely to the challenging requirements of nitride synthesis. Because the triple bond in a nitrogen molecule (N2) makes it very stable, it takes high energy input to break N2 triple bonds and free nitrogen atoms to bond with other atoms. In comparison, the double bond in an oxygen molecule (O2) takes only about half as much energy to break.
So, metals that would nitridize (react with nitrogen) generally oxidize (react with oxygen) instead. Thus, researchers must make sure that nitride synthesis takes place in an oxygen- and water-free atmosphere.
Some scientists have circumvented the N2 triple bond by successfully synthesizing nitrides using nitrogen precursors, such as ammonia, in which nitrogen atoms are not so tightly bound. However, these reactions still require controlled environments to prevent oxidation and also to prevent freed nitrogen atoms from forming N2 molecules.
For these reasons, exploratory synthesis of nitride materials can be a time-consuming and risky venture. To Wenhao Sun, staff scientist at Lawrence Berkeley National Laboratory (LBNL), the materials challenges faced by scientists are similar to challenges faced by world explorers. “Sailing into the unknown was a very risky endeavor, and in the same way, exploration of new chemical spaces can also be risky,” he says in a National Renewable Energy Laboratory (NREL) press release.
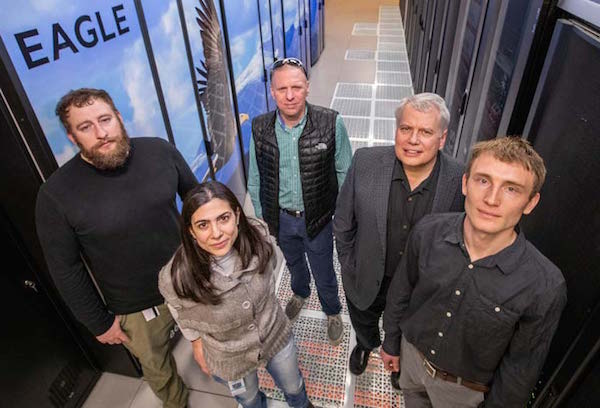
Part of the research team that created a chemical map identifying more than 200 new stable ternary nitride compounds. From left, Aaron Holder, Elisabetta Arca, Stephan Lany, Bill Tumas, and Andriy Zakutayev stand near the Eagle Supercomputer in NREL’s Energy Systems Integration Facility. Credit: Werner Slocum, NREL
However, just like world explorers, Sun says materials scientists have a way to lessen their risk. “Maps help to guide explorers, allowing them to navigate better,” he explains.
And a map is just what he and his colleagues created—a chemical map to guide the exploratory synthesis of nitrides.
The journey to creating a map of ternary metal nitrides started near the end of 2016 with a paper describing the thermodynamic scale of metastability for inorganic crystalline phases. The study showed metastable materials accounted for a significant fraction of nitride compounds, and Sun, who was lead author of the paper, decided a collaboration with NREL would be wise. “NREL has been making metastable nitrides for many years now,” he says.
In 2017, Sun and colleagues from LBNL, NREL, Massachusetts Institute of Technology, and the University of Colorado, Boulder, published a paper describing how they used a data-mined structure-predictor (DMSP) algorithm to predict new metastable binary nitrides.
DMSP, a technique they previously used to identify new stable ternary oxides in 2010, works by making rational chemical substitutions on existing crystal structures to generate new unobserved phases via simulation. Then, “By computing the formation free-energies of DMSP suggested phases in [density functional theory], and then comparing the formation energies of these phases against the known convex hull, we can identify novel stable and stabilizable nitrides,” they explain in the 2017 paper.
In a new study published this month, the researchers along with colleagues from Oregon State University and SLAC National Accelerator Laboratory used DMSP to extrapolate 340 known stable and metastable ternary nitrides to 6,000 hypothetical ternary nitride structures. They then evaluated the phase stability of the DMSP-generated nitrides using ab initio thermodynamics, leveraging tools and precomputed data from The Materials Project database.
Of the 6,000 hypothetical structures, the researchers predicted more than 200 new stable ternary nitride compounds, about double the 213 previously known stable ternary nitrides. Guided by these predictions, they then successfully synthesized seven new zinc- and magnesium-based ternary nitrides.
In an interactive version of the map, metastable ternary nitrides (highlighted green and red) comprise a majority of the surveyed spaces compared to stable ternary nitrides (highlighted blue). Sun says it is important not to discount metastable compounds.
“If you only do materials design with stable materials, your choices are limited. But if you start thinking about which metastable materials can be made, you increase your design space,” he explains in the press release.
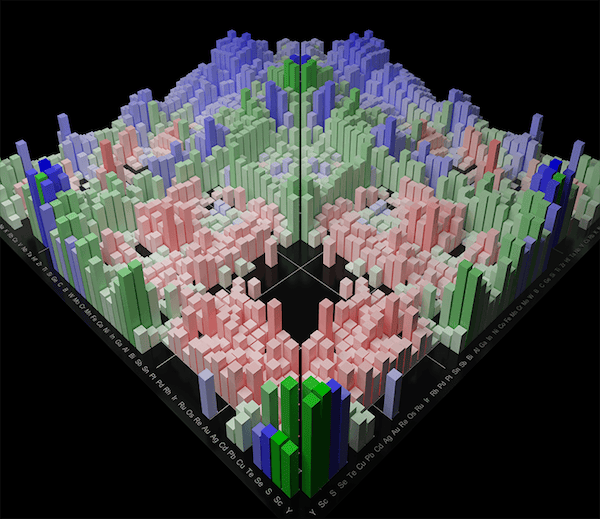
Blue indicates stable ternary nitrides; green indicates ternary nitrides that are metastable against decomposition into binary phases; and red indicates ternary nitrides that are metastable against decomposition into elemental phases. Credit: Josh Bauer, NREL
Creating nitrides is still a time-consuming and risky venture, but with this map, the search for new nitrides can be guided to more fruitful paths.
The 2016 paper on thermodynamic scale of metastability, published in Science Advances, is “The thermodynamic scale of inorganic crystalline metastability” (DOI: 10.1126/sciadv.1600225).
The 2017 paper on binary nitrides, published in Chemistry of Materials, is “Thermodynamic routes to novel metastable nitrogen-rich nitrides” (DOI: 10.1021/acs.chemmater.7b02399).
The 2019 paper on ternary nitrides, published in Nature Materials, is “A map of the inorganic ternary metal nitrides” (DOI: 10.1038/s41563-019-0396-2).
Author
Lisa McDonald
CTT Categories
- Basic Science