What are ceramics and glass?
Broadly speaking, ceramics are nonmetallic, inorganic, crystalline materials. Compounds such as oxides, nitrides, carbides, and borides are generally considered ceramic materials.
On the other hand, glasses are noncrystalline materials with wide composition ranges. However, most commercial glasses are based on silicate or borosilicate compositions.
Despite their microstructural differences, ceramic and glass materials share many properties in common, including being extremely hard, chemically inert, and good thermal insulators. These properties allow ceramic and glass materials to serve in many critical applications, including as a car’s catalytic converter to control harmful exhaust emissions, as optical fibers to enable high-speed communication around the world, and as dental crowns to repair broken teeth.
Everywhere you look, ceramic and glass materials are hard at work—even though they may be unseen. Explore the links below to learn how ceramic and glass materials are used in all sectors of the modern world.
Learn more about ceramics and glass in our world
Want to learn more about the role ceramic and glass materials play in your everyday life? From 2017–2023, the ACerS Bulletin editorial team coordinated a special cover story for the December issue that provided an in-depth look at the ceramic and glass materials used in different areas of your life, including in our homes, bodies, electronics, and more. View all the December cover stories below, and become an ACerS member to access all 100+ years of the ACerS Bulletin!
Learn more about ceramics and glass global markets
Not only are ceramic and glass materials everywhere in our everyday lives, the markets for these materials span the globe as well. Each fall, the October/November issue of the ACerS Bulletin takes a look at these markets in a specific country or continent. Become an ACerS member and access these special market issues of the ACerS Bulletin!
This is ceramics!
Want to learn even more about how ceramics and glass impact our world? Follow ACerS Fellow Ricardo Castro on LinkedIn, where he shares surprising insights about ceramic and glass applications under the hashtag #thisisceramics.
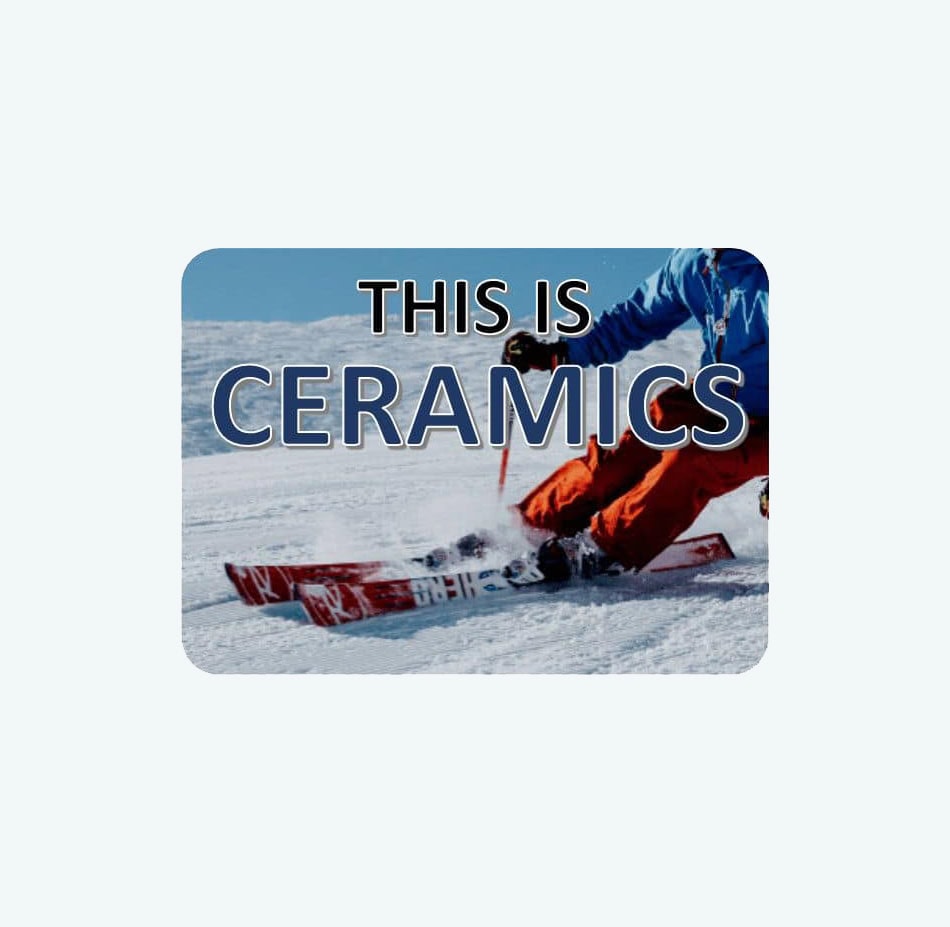
Resources
Want to learn even more about ceramics and glass? The resources below offer a starting point to learn more about these materials!
General materials science resources
Ceramic and glass resources
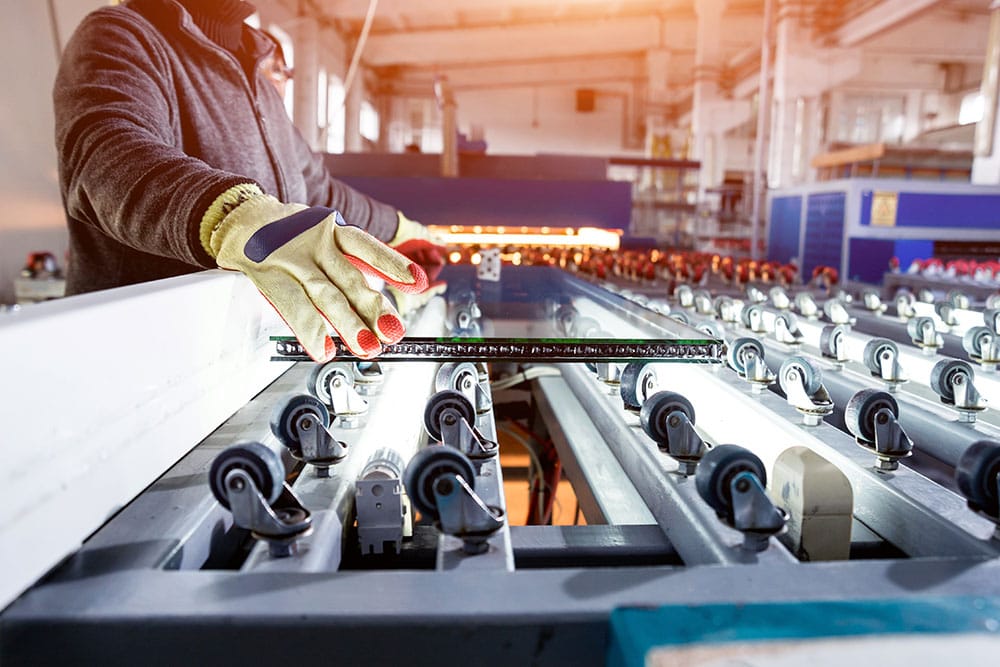
Branches of ceramics
Most people are familiar with traditional ceramics used in everyday objects such as dishes, pottery, sanitaryware, brick, and art. Similarly, we are accustomed to glass in windows, drinking glasses, microscope optics, and fish tanks.
However, advanced ceramics and glass contribute in both obvious and hidden ways. Examples include catalytic converter substrates in vehicles, cover glasses for smartphones and tablets, refractory linings of steelmaking furnaces, fiber optics for communications, bone scaffolds, ceramic armor, cutting tools, and much more.
From better brick to today’s advanced ceramics and glass
Brick manufacturers founded ACerS in 1898 to apply scientific methods to manufacturing of clay products. Today, ACerS members research, develop, manufacture, and design engineered ceramics and glasses for use in nearly every technology.