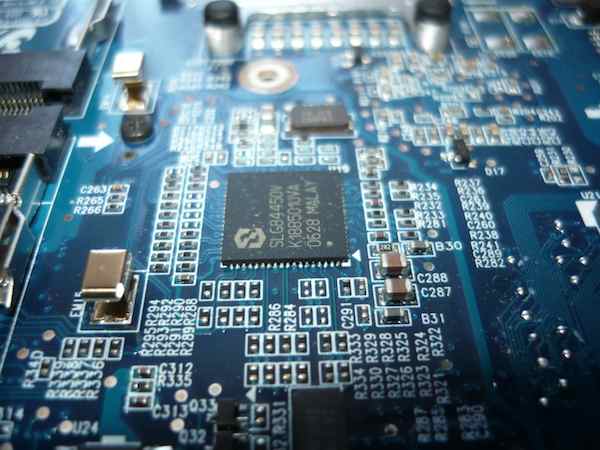
TEM image of a silicon nanowire (NW) encased in an alumina shell (false-colored in blue), which was synthesized by Harvard scientists to improve biomedical nanoelectronic devices. Credit: Lieber lab; Harvard.
Moore’s law has predicted steadily increased capabilities of silicon electronics over the past 50 years, allowing consequent size reductions that have ushered today’s electronics nanorevolution. Everything is nanoscale today—any news feeds reporting the latest scientific advances will include non-nano amounts of the nano prefix.
Nanomedicine is one big way (pun intended) in which nanotechnology may change our lives. Imagine biocompatible nanoelectronics that can be implanted in your body to monitor the presence or changing levels of biomolecules as they happen. Biosensors like these could change our everyday lives—for example, by monitoring and helping to regulate blood sugar levels of a diabetic, detecting preneoplastic biomarkers in cancer-predisposed individuals, or monitoring blood oxygenation levels in performance athletes, to name a very few.
Silicon nanowire nanoelectronics are promising for a wide host of biomedical sensing applications because of their biocompatibility, but problems with extended stability in physiological environments are a speedbump for long-term applications. Now a group from Harvard University has engineered a new approach by encasing silicon nanowires in alumina shells. Their results, published in Nano Letters, show considerable improvements in nanowire stability in preliminary long-term exposure to physiological environments.
“One of the main goals of this work is to allow us to exploit unique spatial-temporal resolution characteristics of nanowire devices for chronic/long-term in vivo studies, which will be very promising for many biomedical and health care applications,” lead authors Wei Zhou and Xiaochuan Dai said in an email. Zhou is a post-doc and Dai is a graduate student in Charles Lieber’s lab.
Using atomic layer deposition, the scientists layered silicon nanowires with 10 nm-thick alumina shells (pictured above) and then tested whether the shells could protect the wires in buffered solutions, including both phosphate-buffered saline (PBS) and cell culture media to mimic the isotonicity and composition of fluids the wires would encounter in the human body.
While naked silicon nanowires exhibit dissolution after just 10 days in body-temperature PBS, adding a 10 nm alumina shell extended nanowire life more than ten times—shelled nanowires showed little dissolution after 100 days. The team saw similar results when the PBS was exchanged for cell culture media, suggesting such longevity may also be achieved in the body.
The researchers went on to test the shelled nanowires’ applicability in field-effect transistors (modeled below), showing the devices had vastly improved stability in warm PBS for at least 4 months.
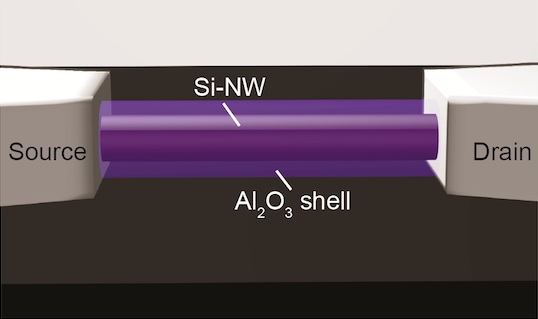
Schematic model of a alumina-encased silicon nanowire (NW) field-effect transistor. Credit: Lieber lab; Harvard.
Alumina shells could similarly extend the lives of non-silicon wires, and the authors further speculate that improving and optimizing the alumina shells may further extend nanowire life.
The next step is to see how well the shells hold up within an intact biological system. “We have already started to work on chronic in vivo electrophysiological studies in rodents using these newly developed core-shell nanowire devices that have improved chemical stability,” Zhou and Dai said in the email. While we’ll have to wait for their results, the future of biomedical nanoelectronics looks promising.
One of the benefits of nanoelectronic devices is the ability to measure biomolecules in real time. For the most part, current medical care is limited to waiting for a problem to occur, after symptoms have developed—and often, after damage has already been done. Once symptoms present, a health care professional has to work backwards to try to determine the underlying problem. Nanoelectronics could reverse that process, by instead detecting the presence or levels of biomolecules in real time and preventing a disturbance in the first place. Not only would this improve individual health, but it would also help reduce staggering healthcare costs.
The paper is “Long Term Stability of Nanowire Nanoelectronics in Physiological Environments” (DOI: 10.1021/nl500070h).
Feature image credit: AAAndrey A; Wikimedia Creative Commons License.