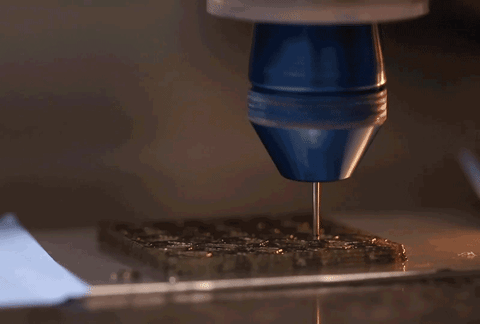
[Image above] A novel 3-D printing method called rotational 3-D printing yields unprecedented control of the arrangement of short fibers embedded in polymer matrices. Credit: Lewis Lab; Harvard SEAS
Nature provides a lot of inspiration to materials scientists and engineers—including puncture resistant gloves modeled after fish scales; 3-D printed polymeric armor inspired by conch shells; glasses that mimic sea sponge spicules; displays modeled after striped mollusks; penguin feather-inspired anti-icing surfaces; and stronger ceramics inspired by hard-knock mollusks. Inspiration is all around, because nature engineers some fascinating multifunctional materials.
When it comes to multifunctional materials, composites are key—because they combine several different types of materials together into one, composites can have unique combinations of properties, such as high stiffness and strength with low weight. Fiber-reinforced composite materials are particularly useful because they combine the strengthening properties of various types of fibers within a polymer matrix.
But even the properties of a composite material itself sometimes aren’t enough to achieve all the performance goals of a particular component—you need to be able to control local microstructure within a component as well. For example, a component may require additional reinforcement in areas that will experience high stress during loading, lessening the likelihood of failure at those points.
And now, it’s possible to do just that using a newly developed additive manufacturing technique for high-performance fiber-reinforced composites.
Researchers at the Harvard John A. Paulson School of Engineering and Applied Sciences (SEAS) have developed a novel 3-D printing technique that adds another dimension of control to additively manufactured composites—local microstructural control.
Called rotational 3-D printing, the method uses a rotating 3-D printer nozzle to control flow of a fiber-reinforced composite ink, locally controlling orientation of fibers with the viscous polymer matrix.
“Being able to locally control fiber orientation within engineered composites has been a grand challenge,” Jennifer A. Lewis, senior author of the study and Hansjorg Wyss Professor of Biologically Inspired Engineering at Harvard SEAS, says in a Harvard news release. “We can now pattern materials in a hierarchical manner, akin to the way that nature builds.”
The Lewis lab is not new to innovation in 3-D printing. Last year, I reported on how the lab’s scientists were whipping up 3-D-printed ceramic foams with dual-level porosity. And a couple of years before that, I reported on how previous lab members had developed the world’s first 3-D electronics printer, called Voxel8.
But rotational 3-D printing can put just the right spin on local microstructural control, additively manufacturing parts with tailored electrical, optical, or thermal properties.
By controlling fiber orientation—whether glass fibers, ceramic whiskers, or metal platelets, for example—within a composite, rotational 3-D printing affords the ability to optimize strength, stiffness, and damage tolerance within a printed component. And, in addition to material versatility, the authors say, the technique is compatible with any extrusion printing method, including fused filament fabrication and direct ink writing.
“Rotational 3-D printing can be used to achieve optimal, or near optimal, fiber arrangements at every location in the printed part, resulting in higher strength and stiffness with less material,” Brett Compton, then-postdoctoral fellow and current mechanical engineering assistant professor at the University of Tennessee, Knoxville, says in the release. “Rather than using magnetic or electric fields to orient fibers, we control the flow of the viscous ink itself to impart the desired fiber orientation.”
Rotational 3-D printing is thus unique in its spatial control during 3-D printing. Engineers can variably control composition within additively manufactured components, tailoring material properties in specific locations—such as flexibility in areas that need to bend or reinforcement in areas that require more rigid structural support.
“One of the exciting things about this work is that it offers a new avenue to produce complex microstructures and to controllably vary the microstructure from region to region,” Jordan Raney, then-postdoctoral fellow and current mechanical engineering assistant and applied mechanics professor of at the University of Pennsylvania says in the release. “More control over structure means more control over the resulting properties, which vastly expands the design space that can be exploited to optimize properties further.”
The paper will soon be published in the Proceeding of the National Academy of Sciences USA.
Did you find this article interesting? Subscribe to the Ceramic Tech Today newsletter to continue to read more articles about the latest news in the ceramic and glass industry! Visit this link to get started.